By definition, a solid material is rigid. For example, if one were to impose shear stress on a solid steel block, the block would not begin to change shape until an extreme amount of stress has been applied. To be more exact, when shear stress is first applied to a rigid material, it deforms slightly but then springs back to its original shape when the stress is relieved.
A plastic material, such as clay, also possesses some degree of rigidity. However, the critical shear stress above which it yields is relatively small, and once this stress is exceeded, the material deforms continuously and irreversibly and does not recover
its original shape when the stress is relieved.
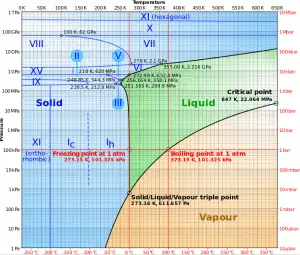
Source: wikipedia.org CC BY-SA
By definition, a fluid material possesses no rigidity at all. For example, if one were to impose shear stress on a fluid element, the fluid element deforms because it cannot withstand any tendency of applied shear stress to change its shape. Furthermore, the more stress that is applied, the more the fluid element will deform. This provides us with a characterizing feature of liquids (and gases—fluids, in general) that distinguishes them from other forms of matter, and we can thus give a formal definition.
There are two types of fluid: liquids and gases. The most important difference between these two types of fluid is in their relative compressibility. Gases can be compressed much more easily than liquids. Consequently, any motion that involves significant pressure variations is generally accompanied by much larger changes in mass density in the case of gas than in the case of a liquid.
Water as a reactor coolant
Water and steam are common fluids used for heat exchange in the primary circuit (from the surface of fuel rods to the coolant flow) and in the secondary circuit. It is used due to its availability and high heat capacity, both for cooling and heating. It is especially effective to transport heat through vaporization and condensation of water because of its very large latent heat of vaporization.
A disadvantage is that water-moderated reactors have to use the high-pressure primary circuit to keep water in the liquid state and achieve sufficient thermodynamic efficiency. Water and steam also react with metals commonly found in industries such as steel and copper, oxidized faster by untreated water and steam. In almost all thermal power stations (coal, gas, nuclear), water is used as the working fluid (used in a closed-loop between boiler, steam turbine, and condenser), and the coolant (used to exchange the waste heat to a water body or carry it away by evaporation in a cooling tower).
Water as a moderator
The neutron moderator, which is important in thermal reactors, is used to moderate, that is, to slow down neutrons from fission to thermal energies. Nuclei with low mass numbers are most effective for this purpose, so the moderator is always a low-mass-number material. Commonly used moderators include regular (light) water (roughly 75% of the world’s reactors), solid graphite (20% of reactors), and heavy water (5% of reactors).
In most nuclear reactors, water is both a coolant and a moderator. The moderation occurs especially on hydrogen nuclei. In the case of the hydrogen (A = 1) as the target nucleus, the incident neutron can be completely stopped – it has the highest average logarithmic energy decrement of all nuclei. On the other hand, hydrogen nuclei have a relatively higher absorption cross-section. Therefore water is not the best moderator according to the moderating ratio.
Water as a neutron shielding
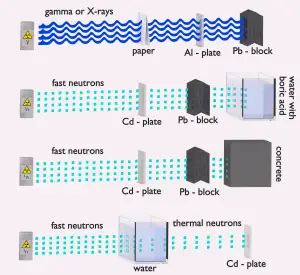
Water, due to the high hydrogen content and availability, is effective and common neutron shielding. However, due to the low atomic number of hydrogen and oxygen, water is not a good shield against gamma rays. On the other hand, in some cases, this disadvantage (low density) can be compensated by the high thickness of the water shield. In the case of neutrons, water perfectly moderates neutrons, but with the absorption of neutrons by hydrogen nuclei, secondary gamma rays with high energy are produced. These gamma rays highly penetrate matter, and therefore they can increase requirements on the thickness of the water shield. Adding a boric acid can help with this problem (neutron absorption on boron nuclei without strong gamma emission) but results in other problems with corrosion of construction materials.
See also: Shielding of Neutrons