Nuclear Physics
What is nuclear physics?
Nuclear physics is the field of physics that studies the constituents of matter (protons and neutrons) and interactions between them. Modern nuclear physics contains especially particle physics, which is taught in close association with nuclear physics.
Key Facts
- The physical world is composed of combinations of various subatomic or fundamental particles. These are the smallest building blocks of matter.
- Fundamental particles and interactions are summarized in a theoretical model called the Standard Model.
- The atoms consist of two parts. An atomic nucleus and an electron cloud.
- The nuclear properties (atomic mass, nuclear cross-sections) of the element are determined by the number of protons (atomic number) and the number of neutrons (neutron number).
- Nuclear stability is a concept that helps to identify the stability of an isotope. It is needed to find the ratio of neutrons to protons to identify the stability of an isotope. To determine the stability of an isotope, you can use the ratio neutron/proton (N/Z).
- Nuclear decay (radioactive decay) occurs when an unstable atom loses energy by emitting ionizing radiation.
- Each type of particle interacts differently. Therefore we must describe the interaction of particles (radiation as a flow of these particles) separately.
- A nuclear reaction is considered to be the process in which two nuclear particles (two nuclei or a nucleus and a nucleon) interact to produce two or more nuclear particles or ˠ-rays (gamma rays).
Atomic and nuclear physics are not the same. The term atomic physics is often associated with nuclear power due to the synonymous use of atomic and nuclear in standard English. However, physicists distinguish between atomic and nuclear physics. Atomic physics deals with the atom as a system consisting of a nucleus and electrons. Nuclear physics deals with the nucleus as a system consisting of nucleons (protons and neutrons). The main difference is in the scale. While the term atomic deals with 1Å = 10-10m, where Å is an ångström (according to Anders Jonas Ångström), the term nuclear deals with 1femtometre = 1fermi = 10-15m.
Atomic Physics
Atomic physics is the field of physics that studies atoms as an isolated system of electrons and an atomic nucleus. It is primarily concerned with the arrangement of electrons around the nucleus and the processes by which these arrangements change. This includes ions and neutral atoms and, unless otherwise stated, it should be assumed that the term atom includes ions for this discussion. Atomic physics also helps to understand the physics of molecules, but molecular physics also describes the physical properties of molecules.
Nuclear Physics
Nuclear physics is the field of physics that studies the constituents (protons and neutrons) and interactions of atomic nuclei. The most commonly known applications of nuclear physics are nuclear power generation, but modern nuclear physics also contains particle physics, which is taught in close association with nuclear physics. Nuclear physics has provided application in many fields, including nuclear medicine (Positron Emission Tomography, isotopes production, etc.) and magnetic resonance imaging, ion implantation in materials engineering, and radiocarbon dating in geology and archaeology.
Our World
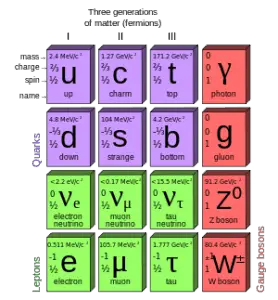
See also: Fundamental Particles.
The physical world is composed of combinations of various subatomic or fundamental particles. These are the smallest building blocks of matter. All matter except dark matter is made of molecules, which are themselves made of atoms. The atoms consist of two parts. An atomic nucleus and an electron cloud. The electrons are spinning around the atomic nucleus. The nucleus itself is generally made of protons and neutrons, but even these are composite objects. Inside the protons and neutrons, we find the quarks.
Quarks and electrons are some of the elementary particles. Several fundamental particles have been discovered in various experiments. So many that researchers had to organize them, just like Mendeleev did with his periodic table. This is summarized in a theoretical model (concerning the electromagnetic, weak, and strong nuclear interactions) called the Standard Model. In particle physics, an elementary particle or fundamental particle is a particle whose substructure is unknown. Thus it is unknown whether it is composed of other particles. Known elementary particles include the fundamental fermions and the fundamental bosons. The fermions are generally “matter particles” and “antimatter particles”.
- Quarks. The quarks combine to form composite particles called hadrons. The best known and most stable are protons and neutrons.
- Antiquarks. For every quark, there is a corresponding type of antiparticle. The antiquarks have the same mass, mean lifetime, and spin as their respective quarks, but the electric charge and other charges have the opposite sign.
- Leptons. The best known of all leptons is the electrons and the neutrinos.
- Antileptons. For every lepton, there is a corresponding type of antiparticle. The best known of all antileptons is the positrons and the antineutrinos.
The bosons are generally “force particles” that mediate interactions among fermions.
- Gauge bosons. The gauge boson is a force carrier of the fundamental interactions of nature.
- Higgs boson. The Higgs bosons give other particles mass via the Higgs mechanism. Their existence was confirmed by CERN on 14 March 2013.
However, only a few of these fundamental particles (some are not fundamental particles) are very important in nuclear engineering. Nuclear engineering or theory of nuclear reactors operates with much better known subatomic particles such as:
- Electrons. The electrons are negatively charged, almost massless particles that nevertheless account for most of the size of the atom. Sir John Joseph Thomson discovered electrons in 1897. Electrons are located in an electron cloud, which is the area surrounding the nucleus of the atom. The electron is only one member of a class of elementary particles, which forms an atom.
- Protons. The protons are positively charged, massive particles that are located inside the atomic nucleus. Ernest Rutherford discovered protons in the year 1919 when he performed his gold foil experiment.
- Neutron. Neutrons are located in the nucleus with protons. Along with protons, they make up almost all of the mass of the atom. Neutrons were discovered by James Chadwick in 1932 when he demonstrated that penetrating radiation incorporated beams of neutral particles.
- Photon. A photon is an elementary particle, the force carrier for the electromagnetic force. The photon is the quantum of light (discrete bundle of electromagnetic energy). Photons are always in motion and, in a vacuum, have a constant speed of light to all observers (c = 2.998 x 108 m/s).
- Neutrino. A neutrino is an elementary particle, one of the particles which make up the universe. Neutrinos are electrically neutral, weakly interacting, and therefore able to pass through great distances in matter without being affected by it.
- Positron. A positron is an antiparticle of a negative electron. Positrons, also called positive electrons, have a positive electric charge and have the same mass and magnitude of charge as the electron. An annihilation occurs when a low-energy positron collides with a low-energy electron.
See also: Fundamental Forces.
Applications of Nuclear Physics
Nuclear physics is the field of physics that studies the constituents of atomic nuclei and interactions of atomic nuclei. Nuclear physics aims to understand the fundamental nuclear forces in nature, their symmetries, and the resulting complex interactions between protons and neutrons in nuclei and among quarks inside hadrons, including the proton.
Nuclear physics is ubiquitous in our lives. Detecting smoke in our homes, testing for and treating cancer, and monitoring cargo for contraband are just some of the ways that nuclear physics and the techniques it has spawned make a difference in our safety, health, and security.
Many of today’s most important advancements in medicine, materials, energy, security, climatology, and dozens of other sciences emanate from the wellspring of basic research and development in nuclear physics.
Nuclear Power
The most commonly known application of nuclear physics is nuclear power generation. Nuclear power can be obtained from nuclear fission, nuclear decay and nuclear fusion reactions. Presently, the vast majority of electricity from nuclear power is produced by nuclear fission of uranium and plutonium in nuclear power plants.
Generating electricity from fusion power remains the focus of international research. Several experimental nuclear fusion reactors and facilities exist. The largest and most ambitious international nuclear fusion project currently in progress is a large tokamak under construction in France.
Nuclear Medicine
Another very important application of nuclear physics is nuclear medicine. Over the past few decades, new nuclear imaging technologies have enhanced the effectiveness of health care and enabled physicians to diagnose different types of cancers, cardiovascular diseases, and neurological disorders in their early stages. The most important modern advances in nuclear imaging are positron emission tomography (PET) and single-photon emission computed tomography (SPECT). Positron emission tomography especially when coupled to X-ray computed tomography scans, has become a highly sensitive probe of abnormal functions.
Magnetic Resonance Imaging
Magnetic resonance imaging was originally called nuclear magnetic resonance imaging, but "nuclear" was dropped to avoid negative associations. Nuclear magnetic resonance is a physical phenomenon in which nuclei in a strong constant magnetic field are perturbed by a weak oscillating magnetic field and respond by producing an electromagnetic signal with a frequency characteristic of the magnetic field at the nucleus. Magnetic resonance imaging is widely used in hospitals and clinics for medical diagnosis, staging and follow-up of disease. Compared to CT scan, magnetic resonance imaging provides better contrast in images of soft-tissues such as in the brain or abdomen.