Nature of Radioactivity
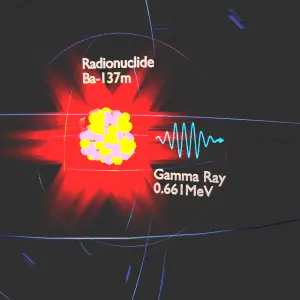
As was written, atomic nuclei consist of protons and neutrons, which attract each other through nuclear force. In contrast, protons repel each other via electromagnetic force due to their positive charge. These two forces compete, leading to various stability of nuclei. There are only certain combinations of neutrons and protons which form stable nuclei. Neutrons stabilize the nucleus because they attract each other and protons, which helps offset the electrical repulsion between protons. As a result, as the number of protons increases, an increasing ratio of neutrons to protons is needed to form a stable nucleus. Suppose there are too many (neutrons also obey the Pauli exclusion principle) or too few neutrons for a given number of protons. In that case, the resulting nucleus is not stable, and it undergoes radioactive decay. Most atoms found in nature are stable and do not emit particles or energy that change form over time. Of the first 82 elements in the periodic table, 80 have isotopes considered stable. Technetium, promethium, and elements with an atomic number over 82 are unstable and decompose through radioactive decay. Unstable isotopes decay spontaneously through various radioactive decay pathways, most commonly alpha decay, beta decay, gamma decay, or electron capture. Many other rare types of decay, such as spontaneous fission or neutron emission, are known.
Decay Modes
Nuclear decay (Radioactive decay) occurs when an unstable atom loses energy by emitting ionizing radiation. Radioactive decay is a random process at the level of single atoms, in that, according to quantum theory, it is impossible to predict when a particular atom will decay. In other words, a nucleus of a radionuclide has no “memory.” A nucleus does not “age” with the passage of time. Thus, the probability of its breaking down does not increase with time but stays constant no matter how long the nucleus has existed. During its unpredictable decay, this unstable nucleus spontaneously and randomly decomposes to form a different nucleus (or a different energy state – gamma decay), giving off radiation in the form of atomic particles or high energy rays. This decay occurs at a constant, predictable rate that is referred to as half-life. A stable nucleus will not undergo this kind of decay and is thus non-radioactive. There are many modes of radioactive decay:
-
Notation of nuclear reactions – radioactive decays
Source: chemwiki.ucdavis.eduAlpha radioactivity. Alpha decay is the emission of alpha particles (helium nuclei). Alpha particles consist of two protons and two neutrons bound together into a particle identical to a helium nucleus. Because of its very large mass (more than 7000 times the mass of the beta particle) and its charge, it heavy ionizes material and has a very short range.
- Beta radioactivity. Beta-decay is the emission of beta particles. Beta particles are high-energy, high-speed electrons or positrons emitted by certain types of radioactive nuclei such as potassium-40. The beta particles have a greater range of penetration than alpha particles but still much less than gamma rays. The beta particles emitted are a form of ionizing radiation, also known as beta rays. The production of beta particles is termed beta decay.
- Gamma radioactivity. Gamma radioactivity consists of gamma rays. Gamma rays are electromagnetic radiation (high energy photons) of a very high frequency and high energy. They are produced by the decay of nuclei as they transition from a high-energy state to a lower state known as gamma decay. Most nuclear reactions are accompanied by gamma emissions.
- Neutron emission. Neutron emission is a type of radioactive decay of nuclei containing excess neutrons (especially fission products), in which a neutron is simply ejected from the nucleus. This type of radiation plays a key role in nuclear reactor control because these neutrons are delayed neutrons.
Conservation Laws in Nuclear Decay
In analyzing nuclear reactions, we apply the many conservation laws. Nuclear reactions are subject to classical conservation laws for a charge, momentum, angular momentum, and energy(including rest energies). Additional conservation laws not anticipated by classical physics are:
- Law of Conservation of Lepton Number
- Law of Conservation of Baryon Number
- Law of Conservation of Electric Charge
Certain of these laws are obeyed under all circumstances, and others are not. We have accepted the conservation of energy and momentum. In all the examples given, we assume that the number of protons and the number of neutrons is separately conserved. We shall find circumstances and conditions in which this rule is not true. Where we are considering non-relativistic nuclear reactions, it is essentially true. However, when considering relativistic nuclear energies or those involving weak interactions, we shall find that these principles must be extended.
Some conservation principles have arisen from theoretical considerations, and others are just empirical relationships. Notwithstanding, any reaction not expressly forbidden by the conservation laws will generally occur, if perhaps at a slow rate. This expectation is based on quantum mechanics. Unless the barrier between the initial and final states is infinitely high, there is always a non-zero probability that a system will make the transition between them.
It is sufficient to note four fundamental laws governing these reactions to analyze non-relativistic reactions.
- Conservation of nucleons. The total number of nucleons before and after a reaction are the same.
- Conservation of charge. The sum of the charges on all the particles before and after a reaction are the same.
- Conservation of momentum. The total momentum of the interacting particles before and after a reaction is the same.
- Conservation of energy. Energy, including rest mass energy, is conserved in nuclear reactions.
Reference: Lamarsh, John R. Introduction to Nuclear engineering 2nd Edition