Beta radiation consists of free electrons or positrons at relativistic speeds. These particles are known as beta particles. Beta particles are high-energy, high-speed electrons or positrons emitted by certain fission fragments or certain primordial radioactive nuclei such as potassium-40. The beta particles are a form of ionizing radiation, also known as beta rays. The production of beta particles is termed beta decay. There are two forms of beta decay:
- the electron decay (β− decay),
- the positron decay (β+ decay).
A nuclear reactor occurs especially the β− decay because the common feature of the fission products is an excess of neutrons (see Nuclear Stability). An unstable fission fragment with the excess of neutrons undergoes β− decay, where the neutron is converted into a proton, an electron, and an electron antineutrino.
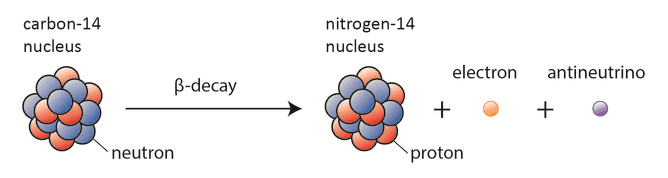
Characteristics of Beta Radiation
Key characteristics of beta radiation are summarized in the following points:
- Beta particles are energetic electrons. They are relatively light and carry a single negative charge.
- Their mass is equal to the mass of the orbital electrons with which they are interacting. A much larger fraction of its kinetic energy can be lost in a single interaction than the alpha particle.
- Their path is not so straightforward. The beta particles follow a very zig-zag path through absorbing material. This resulting path of the particle is longer than the linear penetration (range) into the material.
- Since they have very low mass, beta particles reach mostly relativistic energies.
- Beta particles differ from other heavy charged particles in the fraction of energy lost by the radiative process known as the bremsstrahlung. Therefore for high energy, beta radiation shielding dense materials are inappropriate.
- When the beta particle moves faster than the speed of light (phase velocity) in the material, it generates a shock wave of electromagnetic radiation known as the Cherenkov radiation.
- The beta emission has a continuous spectrum.
- A 1 MeV beta particle can travel approximately 3.5 meters in the air.
- Due to the presence of the bremsstrahlung, low atomic number (Z) materials are appropriate as beta particle shields.
Beta Decay
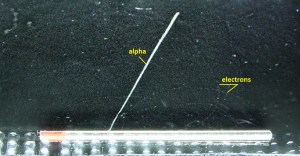
Source: wikipedia.org
Beta-decay or β decay represents the disintegration of a parent nucleus to a daughter through the emission of the beta particle. If a nucleus emits a beta particle, it loses an electron (or positron). In this case, the mass number of the daughter nucleus remains the same, but the daughter nucleus will form a different element. Beta decay is governed by weak interaction. One of two down quarks changes into an up quark during beta decay by emitting a W– boson (carries away a negative charge).
See also: Beta Decay
See also: Detection of Beta Radiation
Beta Radiation – Biological Effects
For photon and electron radiation, the radiation weighting factor has the value 1 independently of the energy of the radiation, and for alpha radiation, the value 20. For neutron radiation, the value is energy-dependent and amounts to 5 to 20. Beta particles (electrons) are much smaller than alpha particles. They carry a single negative charge. They are more penetrating than alpha particles. They can travel several meters but deposit less energy at any point along their paths than alpha particles. This means beta particles tend to damage more cells but with lesser damage to each.
Shielding of Beta Radiation – Electrons
Beta radiation ionizes matter weaker than alpha radiation. On the other hand, the ranges of beta particles are longer and depend strongly on the initial kinetic energy of a particle. Some have enough energy to be of concern regarding external exposure. A 1 MeV beta particle can travel approximately 3.5 meters in the air. Such beta particles can penetrate the body and deposit dose to internal structures near the surface. Therefore greater shielding than in the case of alpha radiation is required.
Materials with low atomic number Z are appropriate as beta particle shields. With high Z materials, the bremsstrahlung (secondary radiation – X-rays) is associated. This radiation is created during the slowing down of beta particles while they travel in a very dense medium. Heavy clothing, thick cardboard, or a thin aluminium plate will protect from beta radiation and prevent of production of the bremsstrahlung.
See also more theory: Interaction of Beta Radiation with Matter
See also calculator: Beta activity to dose rate
Shielding of Beta Radiation – Positrons
The coulomb forces that constitute the major mechanism of energy loss for electrons are present for either positive or negative charge on the particle and constitute the major mechanism of energy loss also for positrons. Whatever the interaction involves a repulsive or attractive force between the incident particle and orbital electron (or atomic nucleus), the impulse and energy transfer for particles of equal mass are about the same. Therefore positrons interact similarly with the matter when they are energetic. The track of positrons in a material is similar to the track of electrons. Even their specific energy loss and range are about the same for equal initial energies.
At the end of their path, positrons differ significantly from electrons. When a positron (antimatter particle) comes to rest, it interacts with an electron (matter particle), resulting in the annihilation of both particles and the complete conversion of their rest mass to pure energy (according to the E=mc2 formula) in the form of two oppositely directed 0.511 MeV gamma rays (photons).
Therefore any positron shield has to include also a gamma-ray shield. A multi-layered radiation shield is appropriate to minimize the bremsstrahlung. Material for the first layer must fulfill the requirements for negative beta radiation shielding. The first layer of such a shield may be a thin aluminium plate (to shield positrons), while the second layer of such a shield may be a dense material such as lead or depleted uranium.
See also: Shielding of Gamma Radiation