In both terms, the neutron generation and the neutron population are closely associated with the multiplication of neutrons in the reactor core. The infinite multiplication factor is the ratio of the neutrons produced by fission in one neutron generation to the number of neutrons lost through absorption in the preceding neutron generation. This can be expressed mathematically, as shown below.
Example:
The following example may well express the difference:
Example:
The number of neutrons (the neutron population) in the core at time zero is 1000 and k∞ = 1.001 (~100 pcm).
Calculate the number of neutrons after 100 generations. Let say the mean generation time is ~0.1s.
Solution:
To calculate the neutron population after 100 neutron generations, we use following equation:
Nn=N0. (k∞)n
N1=N0.1.001 = 1001 neutrons after one generation
N2=N0.1.001.1.001 = 1002 neutrons after two generations
N3=N0.1.001.1.001.1.001 = 1003 neutrons after three generations
.
.
N50=N0. (k∞)50 = 1051 neutrons after fifty generations.
.
.
N100=N0. (k∞)100 = 1105 neutrons after hundred generations.
If we consider the mean generation time to be ~0.1s, the increase from 1000 neutrons to 1105 neutrons occurs within 10 seconds.
The infinite multiplication factor in a multiplying system measures the change in the fission neutron population from one neutron generation to the subsequent generation.
- k∞ < 1. Suppose the multiplication factor for a multiplying system is less than 1.0. In that case, the number of neutrons decreases in time (with the mean generation time), and the chain reaction will never be self-sustaining. This condition is known as the subcritical state.
- k∞ = 1. If the multiplication factor for a multiplying system is equal to 1.0, then there is no change in neutron population in time, and the chain reaction will be self-sustaining. This condition is known as the critical state.
- k∞ > 1. If the multiplication factor for a multiplying system is greater than 1.0, then the multiplying system produces more neutrons than are needed to be self-sustaining. The number of neutrons is exponentially increasing in time (with the mean generation time). This condition is known as the supercritical state.
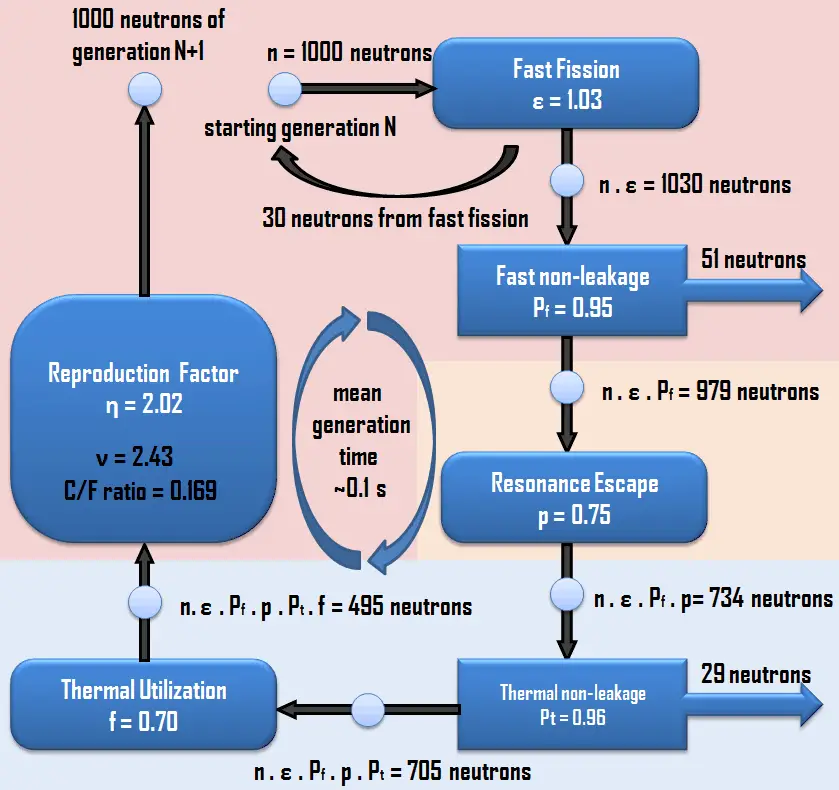
The sequence of fission events is known as the fission chain reaction, and it is important in nuclear reactor physics. The chain reaction can take place only in the proper multiplication environment and only under proper conditions.