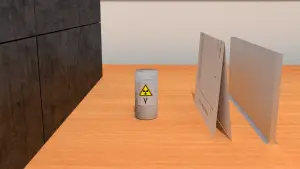
The danger of ionizing radiation lies in the fact that the radiation is invisible and not directly detectable by human senses. People can neither see nor feel radiation, yet it deposits energy into the molecules of the material. The energy is transferred in small quantities for each interaction between the radiation and a molecule, and there are usually many types of interactions. Therefore, the only way you can detect and measure radiation is to use instruments (detectors of ionizing radiation).
Detailed knowledge about the detection of radiation is very important in many branches of engineering, including radiation protection. Most modern nuclear or particle experiments use a variety of sophisticated detectors for measuring and detecting sub-atomic particles. A particle must leave some trace of its presence in a detector to be detected. Particles mostly deposit energy along their path. Knowledge of this interaction, how different particles deposit energy in the matter, and how much energy particles deposit is fundamental for our understanding of many problems. This chapter will give you a basic understanding of how these detectors work and some of their limitations.
Basic Principles of Detectors
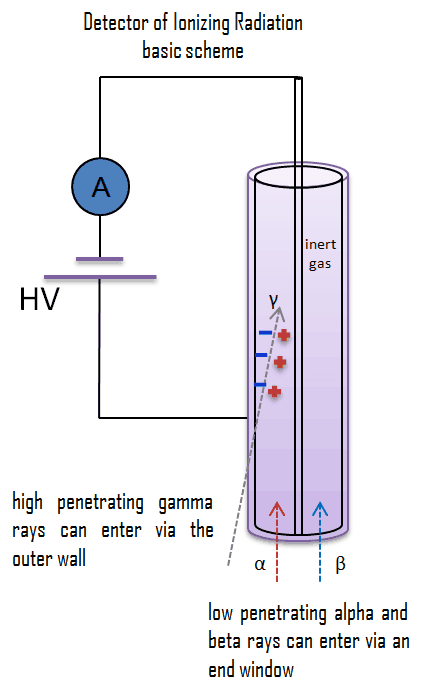
In their basic principles of operation, most detectors of ionizing radiation follow similar characteristics. Detectors of ionizing radiation consist of two parts that are usually connected. The first part consists of sensitive material, consisting of a compound that experiences changes when exposed to radiation. The other component is a device that converts these changes into measurable signals. All detectors require that radiation deposit some of its energy in sensitive material that forms part of the instrument. The radiation enters the detector, interacts with atoms of the detector material, and deposits some energy into sensitive material. Each event may generate a signal, a pulse, hole, light signal, ion pairs in a gas, and many others. The main task is to generate a sufficient signal, amplify it, and record it.
Let’s assume gaseous ionization detectors. A basic gaseous ionization detector consists of a chamber filled with a suitable medium (air or a special fill gas) that can be easily ionized. As a general rule, the center wire is the positive electrode (anode), and the outer cylinder is the negative electrode (cathode), so that (negative) electrons are attracted to the center wire. Positive ions are attracted to the outer cylinder. The anode is at a positive voltage for the detector wall. As ionizing radiation enters the gas between the electrodes, a finite number of ion pairs are formed. Under the influence of the electric field, the positive ions will move toward the negatively charged electrode (outer cylinder), and the negative ions (electrons) will migrate toward the positive electrode (central wire). Collecting these ions will produce a charge on the electrodes and an electrical pulse across the detection circuit. However, it is a small signal. This signal can be amplified and then recorded using standard electronics.
Classification of Radiation Detectors – Purpose
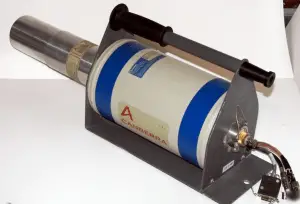
Generally, detectors of ionizing radiation may be classified according to their purpose. Their purpose is closely related to the generated signal in the detector. There are three main types of detectors, which record different types of signals.
- Counter. The activity or intensity of radiation is measured in counts per second (cps). The best-known counter is the Geiger-Müller counter. In radiation counters, the generated signal from the incident radiation is created by counting the number of interactions occurring at the sensitive volume of the detector.
- Radiation Spectrometer. Spectrometers are devices designed to measure the spectral power distribution of a source, and the incident radiation generates a signal that determines the incident particle’s energy.
- Dosimeter. A radiation dosimeter is a device that measures exposure to ionizing radiation. Dosimeters usually record a dose, which is then absorbed radiation energy measured in grays (Gy) of the equivalent dose measured in sieverts (Sv) A personal dosimeter is a dosimeter, that is worn at the surface of the body by the person being monitored, and it records of the radiation dose received.
Classification of Radiation Detectors – Radiation Type
Principles and methods for the detection of ionizing radiation are dependent on many factors. The type of radiation measured and detected is a key factor. Different types of detectors in different physical states (solid, liquid, or gas) are used to measure selective types of ionizing radiation. It is important to distinguish between alpha or beta particles, X-rays or γ-rays, and neutrons. Since each radiation interacts differently with the matter, we cannot use, for example, a neutron detector to detect beta radiation. Sometimes, detectors can detect various types of radiation. For example, neutron detectors are also able to detect gamma radiation. Usually, this feature is not desirable, and detectors must compensate for the undesirable component of radiation.
To describe the classification of radiation detectors by the type of radiation, we must understand the interactions of radiation with matter. Each type of particle interacts in a different way. Therefore we must describe the interaction of particles (radiation as a flow of these particles) separately. For example, charged particles with high energies can directly ionize atoms. On the other hand, electrically neutral particles interact indirectly but can also transfer some or all of their energies to the matter. This is the key feature of the categorization of radiation detectors. We cannot use a gamma rays detector to detect alpha radiation. Detectors can be categorized into two general types as follows:
- Detection of Directly Ionizing Radiation
- Alpha radiation
- Beta radiation
- Detection of Indirectly Ionizing Radiation
- Gamma radiation
- Neutron radiation
- Neutrinos