Also, to help understand this concept, there is a chart of the nuclides, known as a Segre chart. This chart shows a plot of the known nuclides as a function of their atomic and neutron numbers. It can be observed from the chart that there are more neutrons than protons in nuclides with Z greater than about 20 (Calcium). These extra neutrons are necessary for the stability of the heavier nuclei. The excess neutrons act somewhat like nuclear glue.
See also: Live chart – iaea.org
Atomic nuclei consist of protons and neutrons, which attract each other through the nuclear force, while protons repel each other via the electric force due to their positive charge. These two forces compete, leading to various stability of nuclei. There are only certain combinations of neutrons and protons, which form stable nuclei.
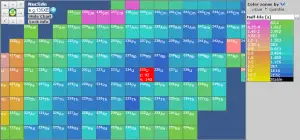
Source: Live chart – IAEA.org
Neutrons stabilize the nucleus because they attract each other and protons, which helps offset the electrical repulsion between protons. As a result, as the number of protons increases, an increasing ratio of neutrons to protons is needed to form a stable nucleus. If there are too many or too few neutrons for a given number of protons, the resulting nucleus is not stable and undergoes radioactive decay. Unstable isotopes decay through various radioactive decay pathways, most commonly alpha decay, beta decay, or electron capture. Many other rare types of decay, such as spontaneous fission or neutron emission, are known. It should be noted that all of these decay pathways may be accompanied by the subsequent emission of gamma radiation. Pure alpha or beta decays are very rare.
Examples:
Nuclear Stability – Periodic Table
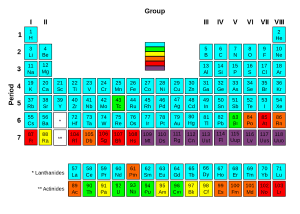
Of the first 82 elements in the periodic table, 80 have isotopes considered to be stable. Technetium, promethium, and all the elements with an atomic number over 82 are unstable and decompose through radioactive decay. No undiscovered heavy elements (with an atomic number over 110) are expected to be stable. Therefore lead is considered the heaviest stable element. For each of the 80 stable elements, the number of stable isotopes is given. For example, tin has 10 such stable isotopes.
There are 80 elements with at least one stable isotope, but 114 to 118 chemical elements are known. All elements to element 98 are found in nature. The remainder of the discovered elements is artificially produced, with isotopes all highly radioactive with relatively short half-lives.
Bismuth, thorium, uranium, and plutonium are primordial nuclides because they have half-lives long enough to be still found on the Earth. In contrast, all the others are produced either by radioactive decay or are synthesized in laboratories and nuclear reactors. Primordial nuclides are nuclides found on the Earth that had existed in their current form since before Earth was formed. Primordial nuclides are residues from the Big Bang, from cosmogenic sources, and from ancient supernova explosions that occurred before the solar system’s formation. Only 288 such nuclides are known.
The connection between Nuclear Stability and Radioactive Decay
The nuclei of radioisotopes are unstable. In an attempt to reach a more stable arrangement of its neutrons and protons, the unstable nucleus will spontaneously decay to form a different nucleus. If the number of neutrons changes in the process (number of protons remains), a different isotope is formed, and an element remains (e.g.,, neutron emission). If the number of protons changes (different atomic numbers) in the process, then an atom of a different element is formed. This decomposition of the nucleus is referred to as radioactive decay. During radioactive decay, an unstable nucleus spontaneously and randomly decomposes to form a different nucleus (or a different energy state – gamma decay), giving off radiation in the form of atomic particles or high-energy rays. This decay occurs at a constant, predictable rate that is referred to as half-life. A stable nucleus will not undergo this kind of decay and is thus non-radioactive.
Pauli Exclusion Principle and Nuclear Stability
The instability of neutron-rich nuclei is given by the Pauli exclusion principle, which states that no two fermions can occupy the same quantum state in a nucleus. If there are significantly more neutrons than protons in a nucleus, some of the neutrons will be higher in energy levels in the nucleus. The filling of all the low energy states is envisioned in the liquid drop model, and that favors the condition A = 2Z. That means equal numbers of protons and neutrons. The Pauli principle also favors even numbers of neutrons and protons: pairs of fermions will be expected to have anti-parallel spin and therefore not contribute to the overall spin.
The Pauli exclusion principle also influences the critical energy of fissile and fissionable nuclei. For example, actinides with odd neutron numbers are usually fissile (fissionable with slow neutrons), while actinides with even neutron numbers are not fissile (but fissionable with fast neutrons). Due to the Pauli exclusion principle, heavy nuclei with an even number of protons and an even number of neutrons are very stable thanks to the occurrence of ‘paired spin.’ On the other hand, nuclei with an odd number of protons and neutrons are mostly unstable.