Detection of alpha radiation is very specific because alpha particles travel only a few centimeters in the air but deposit all their energies along their short paths. Thus the amount of energy transferred is very high.
To describe the principles of detection of alpha radiation, we must understand the interaction of radiation with matter. Each type of particle interacts differently; therefore, we must describe the interaction of alpha particles (radiation as a flow of these particles) separately.
Interaction of Heavy Charged Particles with Matter
Alpha radiation consists of alpha particles at high energy/speed, and the production of alpha particles is termed alpha decay. Alpha particles consist of two protons and two neutrons bound together into a particle identical to a helium nucleus. Alpha particles are relatively large and carry a double positive charge, and they are not very penetrating, and a piece of paper can stop them. In general, heavy charged particles transfer energy mostly by:
- Excitation. The charged particle can transfer energy to the atom, raising electrons to higher energy levels.
- Ionization. Ionization can occur when the charged particle has enough energy to remove an electron, creating ion pairs in surrounding matter.
The distance required to bring the particle to rest referred to as its range. The range of heavy charged particles in solids amounts to only a few microns, and thus most of the energy of these particles is converted to heat very close to the point of its creation. In the case of gases, the range increases to a few centimeters in dependence on gas parameters (density, type of gas, etc.) This distance is very important for detectors and significantly determines the design of all detectors. The trajectory of heavy charged particles in the materials is not greatly affected because they mostly interact with light atomic electrons. Other charged particles, such as the protons, behave similarly with one exception – the ranges are somewhat longer for lighter charged particles.
A convenient variable that describes the ionization properties of the surrounding medium is the stopping power, and the classical expression describing the specific energy loss is the Bethe formula. For alpha particles and heavier particles, the stopping power of most materials is very high for heavy charged particles, and these particles have very short ranges. For example, the range of a 5 MeV alpha particle is approximately only 0,002 cm in aluminium alloy. An ordinary sheet of paper or living tissue can stop most alpha particles.
Detectors of Alpha Radiation
Detectors may also be categorized according to sensitive materials and methods that can be utilized to make a measurement:
Detection of Alpha Radiation using Ionization Chamber
For alpha and beta particles to be detected by ionization chambers, they must be given a thin window. This “end-window” must be thin enough for the alpha and beta particles to penetrate. However, a window of almost any thickness will prevent an alpha particle from entering the chamber. The window is usually made of mica with a density of about 1.5 – 2.0 mg/cm2. But it does not mean an ionization chamber cannot detect alpha radiation.
For example, in some kinds of smoke detectors, you can meet artificial radionuclides such as americium-241, a source of alpha particles. The smoke detector has two ionization chambers, one open to the air and a reference chamber that does not allow the entry of particles. The radioactive source emits alpha particles into both chambers, which ionizes some air molecules. The free-air chamber allows the entry of smoke particles into the sensitive volume and changes the attenuation of alpha particles. If any smoke particles enter the free-air chamber, some ions will attach to the particles and not be available to carry the current in that chamber. An electronic circuit detects that a current difference has developed between the open and sealed chambers and sounds the alarm.
Detection of Alpha Radiation using Geiger-Mueller Counter
Geiger counters are mainly used for portable instrumentation due to their sensitivity, simple counting circuit, and ability to detect low-level radiation. Although the major use of Geiger counters is probably in individual particle detection, they are also found in gamma survey meters. They can detect almost all types of radiation, but there are slight differences in the Geiger-Mueller tube. However, the Geiger-Müller tube produces a pulse output of the same magnitude for all detected radiation, so a Geiger counter with an end window tube cannot distinguish between alpha and beta particles.
End-Window type
For alpha and beta particles to be detected by Geiger counters, they must be given a thin window. This “end-window” must be thin enough for the alpha and beta particles to penetrate. However, a window of almost any thickness will prevent an alpha particle from entering the chamber. The window is usually made of mica with a density of about 1.5 – 2.0 mg/cm2 to allow low-energy beta particles (e.g., from carbon-14) to enter the detector. The efficiency reduction for alpha is due to the attenuation effect of the end window, though the distance from the surface being checked also has a significant effect. Ideally, a source of alpha radiation should be less than 10mm from the detector due to attenuation in the air.
Detection of Alpha using Scintillation Counter
Scintillation counters are used to measure radiation in various applications, including hand-held radiation survey meters, personnel and environmental monitoring for radioactive contamination, medical imaging, radiometric assay, nuclear security, and nuclear plant safety. They are widely used because they can be made inexpensively yet with good efficiency and can measure both the intensity and the energy of incident radiation.
Scintillation counters can be used to detect alpha, beta, and gamma radiation, and they can also be used for the detection of neutrons. For these purposes, different scintillators are used:
Alpha Particles and Heavy Ions. Due to the very high ionizing power of heavy ions, scintillation counters are usually not ideal for detecting heavy ions. For equal energies, a proton will produce 1/4 to 1/2 the light of an electron, while alpha particles will produce only about 1/10 the light. Where needed, inorganic crystals, e.g., CsI(Tl) and ZnS(Ag) (typically used in thin sheets as α-particle monitors), should be preferred to organic materials. Pure CsI is a fast and dense scintillating material with a relatively low light yield that increases significantly with cooling. The drawbacks of CsI are a high-temperature gradient and a slight hygroscopicity.
Detection of Alpha using Semiconductors – Silicon Strip Detectors
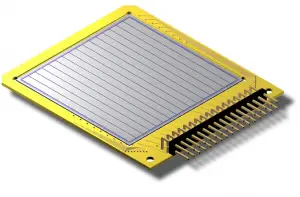
Silicon-based detectors are very good for tracking charged particles. A silicon strip detector is an arrangement of strip-like shaped implants acting as charge-collecting electrodes.
Silicon strip detectors 5 x 5 cm2 in the area are quite common and are used in series (just like planes of MWPCs) to determine charged-particle trajectories to position accuracies of the order of several μm in the transverse direction. These implants form a one-dimensional array of diodes on a low-doped, fully depleted silicon wafer. A position-sensitive detector is built by connecting each metalized strip to a charge-sensitive amplifier. Two-dimensional position measurements can be achieved by applying additional strip-like doping on the wafer backside using double-sided technology. Such devices can measure small impact parameters and thereby determine whether some charged particle originated from a primary collision or was the decay product of a primary particle that traveled a small distance from the original interaction and then decayed.