Pure magnesium is a shiny gray solid that bears a close physical resemblance to the other five elements in the periodic table’s second column (group 2, or alkaline earth metals).
Magnesium alloys are mixtures of magnesium and other alloying metal, usually aluminium, zinc, silicon, manganese, copper, and zirconium. Since the most outstanding characteristic of magnesium is its density, 1.7 g/cm3, its alloys are used where light weight is an important consideration (e.g., in aircraft components). Magnesium has the lowest melting point (923 K (1,202 °F)) of all the alkaline earth metals. Pure magnesium has an HCP crystal structure, is relatively soft, and has a low elastic modulus: of 45 GPa. Magnesium alloys also have a hexagonal lattice structure, which affects the fundamental properties of these alloys. At room temperature, magnesium and its alloys are difficult to work cold because plastic deformation of the hexagonal lattice is more complicated than in cubic latticed metals like aluminium, copper, and steel. Therefore, magnesium alloys are typically used as cast alloys. Despite the reactive nature of the pure magnesium powder, magnesium metal and its alloys have good corrosion resistance.
Aluminium is the most common alloying element. Aluminium, zinc, zirconium, and thorium promote precipitation hardening: manganese improves corrosion resistance, and tin improves castability.
We must add that pure magnesium is highly flammable, especially when powdered or shaved into thin strips, though it is difficult to ignite in mass or bulk. It produces intense, bright, white light when it burns. Flame temperatures of magnesium and some magnesium alloys can reach 3,100°C. Burning or molten magnesium reacts violently with water. Once ignited, such fires are difficult to extinguish because combustion continues in nitrogen (forming magnesium nitride), carbon dioxide (forming magnesium oxide and carbon), and water. Burning magnesium can be quenched using a Class D dry chemical fire extinguisher. Its flammability is greatly reduced by a small amount of calcium in the alloy.
Uses of Magnesium Alloys – Application
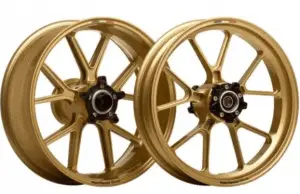
Magnesium alloys are used in a wide variety of structural and nonstructural applications. Structural applications include automotive, industrial, materials handling, commercial, and aerospace equipment. Magnesium alloys are used for parts that operate at high speeds and thus must be lightweight to minimize inertial forces. Commercial applications include hand-held tools, laptops, luggage, and ladders, automobiles (e.g., steering wheels and columns, seat frames, transmission cases). Magnox (alloy), an abbreviation for “magnesium non-oxidizing,” is 99% magnesium and 1% aluminum, and is used in the cladding of fuel rods in magnox nuclear power reactors.
Types of Magnesium Alloys
Magnesium alloys’ names are often given by two letters followed by two numbers. Letters tell main alloying elements (A = aluminium, Z = zinc, M = manganese, S = silicon). Numbers indicate respective nominal compositions of main alloying elements. For example, alloy AZ81 is magnesium alloy with roughly 8% of aluminium and 1% of zinc.
Magnox Alloy – AL80
Magnox, an abbreviation for “magnesium non-oxidizing,” is a high magnesium alloy mainly of magnesium with small amounts of aluminium and other metals. This alloy, designated as alloy AL80, is used as a clad for nuclear fuel employed in the UK gas-cooled, graphite-moderated power station reactors of the same name. The claddings from magnesium alloys of type Magnox are well compatible with metallic uranium at temperatures up to 500°C and are highly resistant to oxidation.
This material has the advantage of a low neutron capture cross-section but has two major disadvantages:
- It limits the maximum temperature (to about 415°C), and hence the thermal efficiency, of the plant.
- The disadvantage of these alloys is a high tendency to grain growth, loss of strength properties, and oxidation in the presence of water steam.
The magnox alloy Al80 has a composition of 0.8% aluminium and 0.004% beryllium.
As was written, magnox alloy AL80 was used primarily as the fuel clad in Magnox-type reactors. On 30 December 2015, Wylfa Unit 1, the world’s last operating Magnox reactor, was closed. As the Magnox design was being rolled out, work was already underway on the Advanced Gas-cooled Reactor (AGR) with the explicit intention of making the system more economical. The AGR design retained Magnox’s graphite moderator and carbon dioxide coolant but increased the cooling gas operating temperature to improve steam conditions. This increased temperature (about 650 °C (1,202 °F)) would greatly improve the thermal efficiency of the power plant. But this was too hot for the magnox alloy, and the AGR originally intended to use a new beryllium-based cladding, but this proved too brittle. This was replaced by a stainless steel cladding. However, steel has a higher neutron cross-section, and this change required the use of slightly enriched uranium fuel to compensate for higher absorption cross-sections.
Elektron 21 – UNS M12310
Elektron is the registered trademark of a wide range of magnesium alloys manufactured by a British company Magnesium Elektron Limited. Elektron 21, designated by UNS M12310, is one of the alloys with excellent corrosion resistance and castability. Cast products possess a fine-grained microstructure and pressure tightness, and this alloy can be easily machined. The application includes motorsports and aerospace since it possesses high strength, is lightweight, and has excellent vibration damping characteristics.
Properties of Magnesium Alloys
Material properties are intensive properties, which means they are independent of the amount of mass and may vary from place to place within the system at any moment. Materials science involves studying materials’ structure and relating them to their properties (mechanical, electrical, etc.). Once materials scientist knows about this structure-property correlation, they can then go on to study the relative performance of a material in a given application. The major determinants of a material’s structure and, thus, its properties are its constituent chemical elements and how it has been processed into its final form.
Mechanical Properties of Magnesium Alloys
Materials are frequently chosen for various applications because they have desirable combinations of mechanical characteristics. For structural applications, material properties are crucial, and engineers must consider them.
Strength of Magnesium Alloys
In the mechanics of materials, the strength of a material is its ability to withstand an applied load without failure or plastic deformation. The strength of materials considers the relationship between the external loads applied to a material and the resulting deformation or change in material dimensions. The strength of a material is its ability to withstand this applied load without failure or plastic deformation.
Ultimate Tensile Strength
Ultimate tensile strength of Elektron 21 – UNS M12310 is about 280 MPa.
The ultimate tensile strength is the maximum on the engineering stress-strain curve. This corresponds to the maximum stress sustained by a structure in tension. Ultimate tensile strength is often shortened to “tensile strength” or “the ultimate.” If this stress is applied and maintained, a fracture will result. Often, this value is significantly more than the yield stress (as much as 50 to 60 percent more than the yield for some types of metals). When a ductile material reaches its ultimate strength, it experiences necking where the cross-sectional area reduces locally. The stress-strain curve contains no higher stress than the ultimate strength. Even though deformations can continue to increase, the stress usually decreases after achieving the ultimate strength. It is an intensive property; therefore, its value does not depend on the size of the test specimen. However, it depends on other factors, such as the specimen preparation, the presence or otherwise of surface defects, and the temperature of the test environment and material. Ultimate tensile strengths vary from 50 MPa for aluminum to as high as 3000 MPa for very high-strength steels.
Yield Strength
Yield strength of Elektron 21 – UNS M12310 is about 145 MPa.
The yield point is the point on a stress-strain curve that indicates the limit of elastic behavior and the beginning plastic behavior. Yield strength or yield stress is the material property defined as the stress at which a material begins to deform plastically. In contrast, the yield point is where nonlinear (elastic + plastic) deformation begins. Before the yield point, the material will deform elastically and return to its original shape when the applied stress is removed. Once the yield point is passed, some fraction of the deformation will be permanent and non-reversible. Some steels and other materials exhibit a behavior termed a yield point phenomenon. Yield strengths vary from 35 MPa for low-strength aluminum to greater than 1400 MPa for high-strength steel.
Young’s Modulus of Elasticity
Young’s modulus of elasticity of Elektron 21 – UNS M12310 is about 45 GPa.
Young’s modulus of elasticity is the elastic modulus for tensile and compressive stress in the linear elasticity regime of a uniaxial deformation and is usually assessed by tensile tests. Up to limiting stress, a body will be able to recover its dimensions on the removal of the load. The applied stresses cause the atoms in a crystal to move from their equilibrium position, and all the atoms are displaced the same amount and maintain their relative geometry. When the stresses are removed, all the atoms return to their original positions, and no permanent deformation occurs. According to Hooke’s law, the stress is proportional to the strain (in the elastic region), and the slope is Young’s modulus. Young’s modulus is equal to the longitudinal stress divided by the strain.
The hardness of Magnesium Alloys
Brinell hardness of Elektron 21 – UNS M12310 is approximately 70 HB.
Rockwell hardness test is one of the most common indentation hardness tests developed for hardness testing. In contrast to the Brinell test, the Rockwell tester measures the depth of penetration of an indenter under a large load (major load) compared to the penetration made by a preload (minor load). The minor load establishes the zero position, and the major load is applied, then removed while maintaining the minor load. The difference between the penetration depth before and after applying the major load is used to calculate the Rockwell hardness number. That is, the penetration depth and hardness are inversely proportional. The chief advantage of Rockwell hardness is its ability to display hardness values directly. The result is a dimensionless number noted as HRA, HRB, HRC, etc., where the last letter is the respective Rockwell scale.
The Rockwell C test is performed with a Brale penetrator (120°diamond cone) and a major load of 150kg.
Thermal Properties of Magnesium Alloys
Thermal properties of materials refer to the response of materials to changes in their temperature and the application of heat. As a solid absorbs energy in the form of heat, its temperature rises, and its dimensions increase. But different materials react to the application of heat differently.
Heat capacity, thermal expansion, and thermal conductivity are often critical in solids’ practical use.
Melting Point of Magnesium Alloys
Melting point of Elektron 21 – UNS M12310 is around 550 – 640°C.
In general, melting is a phase change of a substance from the solid to the liquid phase. The melting point of a substance is the temperature at which this phase change occurs. The melting point also defines a condition where the solid and liquid can exist in equilibrium.
Thermal Conductivity of Magnesium Alloys
The thermal conductivity of Elektron 21 – UNS M12310 is 116 W/(m.K).
The heat transfer characteristics of solid material are measured by a property called the thermal conductivity, k (or λ), measured in W/m.K. It measures a substance’s ability to transfer heat through a material by conduction. Note that Fourier’s law applies to all matter, regardless of its state (solid, liquid, or gas). Therefore, it is also defined as liquids and gases.
The thermal conductivity of most liquids and solids varies with temperature, and for vapors, it also depends upon pressure. In general:
Most materials are nearly homogeneous. Therefore we can usually write k = k (T). Similar definitions are associated with thermal conductivities in the y- and z-directions (ky, kz). However, for an isotropic material, the thermal conductivity is independent of the transfer direction, kx = ky = kz = k.