Article Summary & FAQs
What is ionizing radiation?
Ionizing radiation comprises particles or electromagnetic waves that create the ionizing effect. The kinetic energy of particles (photons, electrons, etc.) of ionizing radiation is sufficient, and the particle can ionize (to form ions by losing electrons) and target atoms to form ions.
Key Facts
- Ionizing radiation has different ionization mechanisms and may be grouped as follows:
- Directly ionizing. Charged particles (atomic nuclei, electrons, positrons, protons, muons, etc.) can ionize atoms directly by fundamental interaction through the Coulomb force if it carries sufficient kinetic energy.
- Alpha radiation. Alpha radiation consists of alpha particles at high energy/speed. The production of alpha particles is termed alpha decay.
- Beta radiation. Beta radiation consists of free electrons or positrons at relativistic speeds. The production of beta particles is termed beta decay.
- Indirectly ionizing. Indirect ionizing radiation is electrically neutral particles and therefore does not interact strongly with matter.
- Photon radiation (Gamma rays or X-rays). Photon radiation consists of high-energy photons. According to the currently valid definition, X-rays are emitted by electrons outside the nucleus, while gamma rays are emitted by the nucleus. The production of gamma rays is termed gamma decay.
- Neutron radiation. Neutron radiation consists of free neutrons at any energy/speed. Nuclear reactors or in-flight can produce this type of radiation, and neutrons contribute 40 – 80% of the equivalent dose.
- Directly ionizing. Charged particles (atomic nuclei, electrons, positrons, protons, muons, etc.) can ionize atoms directly by fundamental interaction through the Coulomb force if it carries sufficient kinetic energy.
- There are three main types of radiation detectors, which record different types of signals.
- Counter. The activity or intensity of radiation is measured in counts per second (cps).
- Radiation Spectrometer. Spectrometers are devices designed to measure the spectral power distribution of a source.
- Dosimeter. A radiation dosimeter is a device that measures exposure to ionizing radiation.
- In general, there are two broad categories of radiation sources:
- Natural Background Radiation. Natural background radiation includes radiation produced by the Sun, lightning, primordial radioisotopes or supernova explosions, etc.
- Man-Made Sources of Radiation. Manufactured sources include medical uses of radiation, residues from nuclear tests, industrial uses of radiation, etc.
Ionizing radiation is any radiation (particles or electromagnetic waves) that carries enough energy to knock electrons from atoms or molecules, thereby ionizing them. For ionizing radiation, the kinetic energy of particles (photons, electrons, etc.) is sufficient, and the particle can ionize (to form ions by losing electrons) to target atoms to form ions.
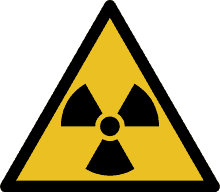
The boundary between ionizing and non-ionizing radiation is not sharply defined since different molecules and atoms ionize at different energies. This is typical for electromagnetic waves. Among electromagnetic waves belong in the order of increasing frequency (energy) and decreasing wavelength: radio waves, microwaves, infrared radiation, visible light, ultraviolet radiation, X-rays, and gamma rays. Gamma rays, X-rays, and the higher ultraviolet part of the spectrum are ionizing, whereas the lower ultraviolet, visible light (including laser light), infrared, microwaves, and radio waves are considered non-ionizing radiation.
All biological damage effects begin with the consequence of radiation interactions with the atoms forming the cells. All living things are composed of one or more cells, and every part of your body consists of cells or was built by them. Although we tend to think of biological effects in terms of the effect of radiation on living cells, in actuality, ionizing radiation, by definition, interacts only with atoms by a process called ionization.
The danger of ionizing radiation lies in the fact that the radiation is invisible and not directly detectable by human senses. People can neither see nor feel radiation, yet it deposits energy into the body’s molecules. The energy is transferred in small quantities for each interaction between the radiation and a molecule, and there are usually many such interactions.
Forms of ionizing radiation
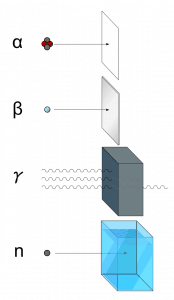
Ionizing radiation is categorized by the nature of the particles or electromagnetic waves that create the ionizing effect. These particles/waves have different ionization mechanisms and may be grouped as:
- Directly ionizing. Charged particles (atomic nuclei, electrons, positrons, protons, muons, etc.) can ionize atoms directly by fundamental interaction through the Coulomb force if it carries sufficient kinetic energy. These particles must be moving at relativistic speeds to reach the required kinetic energy. Even photons (gamma rays and X-rays) can ionize atoms directly (despite they are electrically neutral) through the Photoelectric effect and the Compton effect, but secondary (indirect) ionization is much more significant.
- Alpha radiation. Alpha radiation consists of alpha particles at high energy/speed. The production of alpha particles is termed alpha decay. Alpha particles consist of two protons and two neutrons bound together into a particle identical to a helium nucleus. Alpha particles are relatively large and carry a double positive charge. They are not very penetrating, and a piece of paper can stop them. They travel only a few centimeters but deposit all their energies along their short paths.
- Beta radiation. Beta radiation consists of free electrons or positrons at relativistic speeds. Beta particles (electrons) are much smaller than alpha particles and carry a single negative charge. They are more penetrating than alpha particles, but thin aluminum metal can stop them. They can travel several meters but deposit less energy at any point along their paths than alpha particles.
- Indirectly ionizing. Indirect ionizing radiation is electrically neutral particles and therefore does not interact strongly with matter. The bulk of the ionization effects is due to secondary ionizations.
- Photon radiation (Gamma rays or X-rays). Photon radiation consists of high-energy photons. These photons are particles/waves (Wave-Particle Duality) without rest mass or electrical charge. They can travel 10 meters or more in the air, which is a long distance compared to alpha or beta particles. However, gamma rays deposit less energy along their paths. Lead, water, and concrete stop gamma radiation. Photons (gamma rays and X-rays) can ionize atoms directly through the Photoelectric effect and the Compton effect, where the relatively energetic electron is produced. The secondary electron will go on to produce multiple ionization events; therefore, the secondary (indirect) ionization is much more significant.
- Neutron radiation. Neutron radiation consists of free neutrons at any energy/speed. Neutrons can be emitted by nuclear fission or by the decay of some radioactive atoms. Neutrons have zero electrical charges and cannot directly cause ionization, and neutrons ionize matter only indirectly. For example, proton radiation (fast protons) results when neutrons strike the hydrogen nuclei. Neutrons can range from high-speed, high-energy particles to low-speed, low-energy particles (called thermal neutrons). Neutrons can travel hundreds of feet in the air without any interaction.
High-LET and Low-LET Radiation
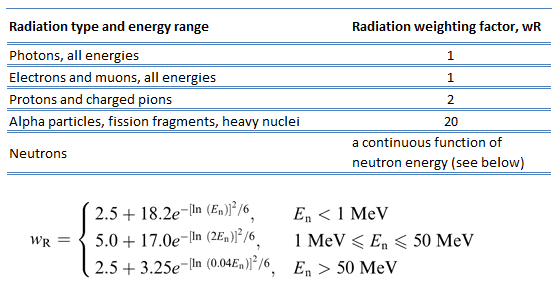
As was written, each type of radiation interacts with matter in a different way. For example, charged particles with high energies can directly ionize atoms. Alpha particles are fairly massive and carry a double positive charge, so they tend to travel only a short distance and do not penetrate very far into a tissue, if at all. However, alpha particles will deposit their energy over a smaller volume (possibly only a few cells if they enter a body) and cause more damage to those few cells.
Beta particles (electrons) are much smaller than alpha particles and carry a single negative charge. They are more penetrating than alpha particles and can travel several meters but deposit less energy at any point along their paths than alpha particles. This means beta particles tend to damage more cells but with lesser damage. On the other hand, electrically neutral particles interact indirectly but can also transfer some or all of their energies to the matter.
It would certainly simplify matters if the biological effects of radiation were directly proportional to the absorbed dose. Unfortunately, biological effects also depend on how the absorbed dose is distributed along the radiation path. Studies have shown that alpha and neutron radiation cause greater biological damage for a given energy deposition per kg of tissue than gamma radiation does. Biological effects of any radiation increase with the linear energy transfer (LET) were discovered. In short, the biological damage from high-LET radiation (alpha particles, protons, or neutrons) is much greater than that from low-LET radiation (gamma rays). This is because the living tissue can more easily repair damage from radiation spread over a large area than concentrated in a small area. Of course, at very high levels of exposure, gamma rays can still cause a great deal of damage to tissues.
Because more biological damage is caused for the same physical dose (i.e., the same energy deposited per unit mass of tissue), one gray of alpha or neutron radiation is more harmful than one gray of gamma radiation. The fact that radiations of different types (and energies) give different biological effects for the same absorbed dose is described in terms of factors known as the relative biological effectiveness (RBE) and the radiation weighting factor (wR).
The radiation weighting factor is a dimensionless factor used to determine the equivalent dose from the absorbed dose averaged over a tissue or organ based on the type of radiation absorbed. The resulting weighted dose was designated as the organ- or tissue equivalent dose:
An equivalent dose of one Sievert represents that quantity of radiation dose equivalent to specified biological damage to one gray of X-rays or gamma rays. An equivalent dose is a non-physical quantity (wR is derived from biological consequences of ionizing radiation) widely used in dosimetry measured by dosimeters. The ICRP designates an equivalent dose as a “limiting quantity”; to specify exposure limits to ensure that “the occurrence of stochastic health effects is kept below unacceptable levels and those tissue reactions are avoided.”
Ionization Energy
Ionization energy, also called ionization potential, is the energy necessary to remove an electron from the neutral atom.
X + energy → X+ + e−
where X is any atom or molecule capable of being ionized, X+ is that atom or molecule with an electron removed (positive ion), and e− is the removed electron.
A nitrogen atom, for example, requires the following ionization energy to remove the outermost electron.
N + IE → N+ + e− IE = 14.5 eV
The ionization energy associated with removing the first electron is most commonly used. The nth ionization energy refers to the amount of energy required to remove an electron from the species with a charge of (n-1).
1st ionization energy
X → X+ + e−
2nd ionization energy
X+ → X2+ + e−
3rd ionization energy
X2+ → X3+ + e−
Ionization Energy for different Elements
There is ionization energy for each successive electron removed. The electrons that circle the nucleus move in fairly well-defined orbits. Some of these electrons are more tightly bound in the atom than others. For example, only 7.38 eV is required to remove the outermost electron from a lead atom, while 88,000 eV is required to remove the innermost electron. Helps to understand the reactivity of elements (especially metals, which lose electrons).
In general, the ionization energy increases moving up a group and moving left to the right across a period. Moreover:
- Ionization energy is lowest for the alkali metals, which have a single electron outside a closed shell.
- Ionization energy increases across a row on the periodic maximum for the noble gases, which have closed shells.
For example, sodium requires only 496 kJ/mol or 5.14 eV/atom to ionize it. On the other hand, neon, the noble gas immediately preceding it in the periodic table, requires 2081 kJ/mol or 21.56 eV/atom.
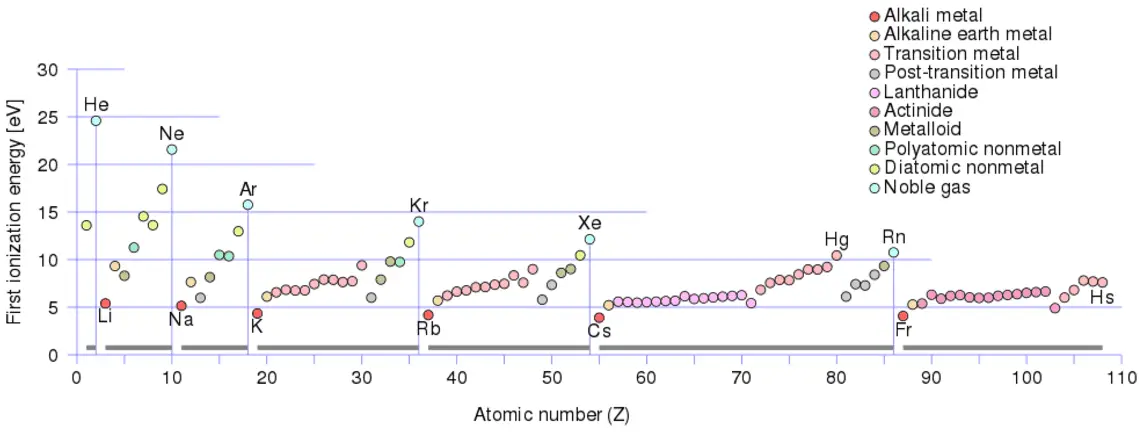