Radiation is all around us. In, around, and above the world we live in. It is a natural energy force that surrounds us, and it is a part of our natural world that has been here since the birth of our planet. From the beginning of time, all living creatures have been, and are still being, exposed to ionizing radiation. Ionizing radiation is generated through nuclear reactions, nuclear decay, very high temperature, or acceleration of charged particles in electromagnetic fields.
Natural Background Radiation
Natural background radiation is ionizing radiation that originates from various natural sources. From the beginning of time, all living creatures have been, and are still being, exposed to ionizing radiation. This radiation is not associated with any human activity. Radioactive isotopes exist in our bodies, houses, air, water, and soil. We all are also exposed to radiation from outer space.
Sources of Natural Background Radiation
We divide all these natural radiation sources into three groups:
Cosmic Radiation
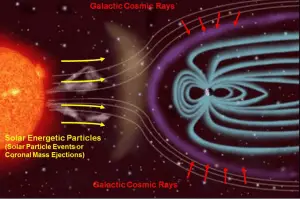
Cosmic radiation refers to sources of radiation in the form of cosmic rays that come from the sun or outer space. At ground level, the muons, with energies mostly between 1 and 20 GeV, contribute about 75 % of the absorbed dose rate in free air. The remainder comes from electrons produced by the muons or present in the electromagnetic cascade. The annual cosmic ray dose at sea level is around 0.27 mSv (27 mrem). If you live at higher elevations or are a frequent airline passenger, this exposure can be significantly higher since the atmosphere is thinner here. The effects of the Earth’s magnetic field also determine the dose of cosmic radiation.
Cosmic radiation can be divided into different types according to its origin. There are three main sources of such radiation:
- Solar Cosmic Radiation. Solar cosmic radiation refers to sources of radiation in the form of high-energy particles (predominantly protons) emitted by the sun, primarily in solar particle events (SPEs).
- Galactic Cosmic Radiation. Galactic cosmic radiation, GCR, refers to sources of radiation in the form of high-energy particles originating outside the solar system but generally from within our Milky Way galaxy.
- Radiation from Earth’s Radiation Belts (van Allen belts). Van Allen radiation belts are zones of high-energy particles (especially protons) trapped by Earth’s magnetic field.
Natural Background in Airplane – Radiation in Flight
Exposure to cosmic radiation increases rapidly with altitude. In-flight, there are two principal sources of natural radiation to consider: Galactic Cosmic Rays, which are always present, and Solar Proton Events, sometimes called Solar Cosmic Ray (SCR) events, which occur sporadically. The dose rate from cosmic radiation varies in different parts of the world and depends strongly on the geomagnetic field, altitude, and solar cycle. The radiation field at aircraft altitudes consists of neutrons, protons, and pions. In-flight, neutrons contribute 40 – 80% of the equivalent dose, depending on the geomagnetic field, altitude, and solar cycle. The cosmic radiation dose rate on airplanes is so high (but not dangerous) that, according to the United Nations UNSCEAR 2000 Report, airline flight crew workers receive more dose on average than any other worker, including those in nuclear power plants.
The ground level dose rate is, on average, about 0.10 μSv/h, but at the maximum flight altitude (8.8 km or 29,000 ft), it can reach about 2.0 μSv/h (or even higher values). A dose rate of 4 μSv/h may represent the average dose rate for all long-haul flights (due to higher altitudes). It must be added that for supersonic planes like the Concorde, which could make a transatlantic flight in 3.5 hours, the exposure rate (about 9 μSv/h) at their altitude of 18 km was increased enough to result in the same cosmic ray exposure per crossing as for conventional jets trundling along at about 8 km.
Earth’s Magnetic Field as Radiation Shield
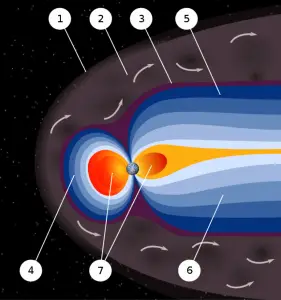
Earth’s magnetic field provides a vital radiation shield from cosmic radiation. In addition to a protective atmosphere, we are lucky that Earth has a magnetic field. The magnetic field extends several tens of thousands of kilometers into space, protecting the Earth from the charged particles of the solar wind and cosmic rays that would otherwise strip away the upper atmosphere, including the ozone layer that protects the Earth from harmful ultraviolet radiation. It shields us from the full effects of solar wind and GCR. Without this protection, Earth’s biosphere might not exist as it does today or would be at least limited to the subsurface. Earth’s magnetic field also provides a radiation shield for astronauts and the ISS because it is in low Earth orbit.
Calculations of the loss of carbon dioxide from the atmosphere of Mars, resulting from the scavenging of ions by the solar wind, indicate that the dissipation of the magnetic field of Mars caused a near-total loss of its atmosphere.
Terrestrial Radiation
Terrestrial radiation refers to soil, water, and vegetation radiation sources. The major isotopes of concern for terrestrial radiation are uranium and the decay products of uranium, such as thorium, radium, and radon. The average dose rate that originates from terrestrial nuclides (except radon exposure) is about 0.057 µGy/hr. The maximum values have been measured on monazite sand in Guarapari, Brazil (up to 50 µGy/hr and in Kerala, India (about 2 µGy/hr), and on rocks with a high radium concentration in Ramsar, Iran (from 1 to 10 µGy/hr).
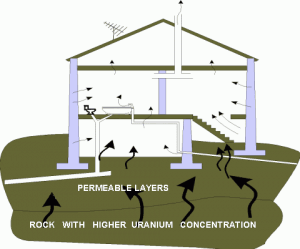
The average annual radiation dose to a person from radon is about 2 mSv/year, which may vary over many orders of magnitude from place to place. Radon is so important that it is usually treated separately. Radon is a colorless, odorless, tasteless noble gas that seeps continuously from bedrock but can accumulate in poorly ventilated houses because of its high density. The fact radon is gas plays a crucial role in the spreading of all its daughter nuclei. Simply radon is a transport medium from bedrock to the atmosphere (or inside buildings) for its short-lived decay products (Pb-210 and Po-210), which posses much more health risks.
Internal Radiation
In addition to the cosmic and terrestrial sources, all people also have radioactive potassium-40, carbon-14, lead-210, and other isotopes inside their bodies from birth.
These isotopes are especially potassium-40, carbon-14, and the isotopes of uranium and thorium. The variation in radiation dose from one person to another is not as great as the variation in dose from cosmic and terrestrial sources. The average annual radiation dose to a person from internal radioactive materials other than radon is about 0.3 mSv/year which:
- 0.2 mSv/year comes from potassium-40,
- 0.12 mSv/year comes from the uranium and thorium series,
- 12 μSv/year comes from carbon-40.
Background Radiation and Health Hazard
You can not go through life without radiation. The danger of ionizing radiation lies in the fact that the radiation is invisible and not directly detectable by human senses. People can neither see nor feel radiation, yet it deposits energy into the molecules of the body.
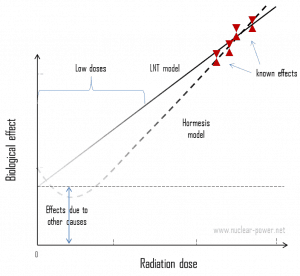
But don’t worry. The doses from background radiation are usually very small (except for radon exposure). A low dose means additional small doses comparable to the normal background radiation (10 µSv = average daily dose received from natural background). The problem is that, at very low doses, it is practically impossible to correlate any irradiation with certain biological effects. This is because the baseline cancer rate is already very high, and the risk of developing cancer fluctuates by 40% because of individual lifestyle and environmental effects, obscuring the subtle effects of low-level radiation.
Secondly, and this is crucial, the truth about low-dose radiation health effects still needs to be found. It is unknown whether these low doses of radiation are detrimental or beneficial (and where is the threshold). Government and regulatory bodies assume an LNT model instead of a threshold or hormesis not because it is the more scientifically convincing but because it is, the more conservative estimate. The problem with this model is that it neglects many defense biological processes that may be crucial at low doses. The research during the last two decades is very interesting and shows that small doses of radiation given at a low dose rate stimulate the defense mechanisms. Therefore the LNT model is not universally accepted, with some proposing an adaptive dose-response relationship where low doses are protective, and high doses are detrimental. Many studies have contradicted the LNT model, and many have shown adaptive response to low dose radiation resulting in reduced mutations and cancers. This phenomenon is known as radiation hormesis.
According to the radiation hormesis hypothesis, radiation exposure comparable to and just above the natural background level of radiation is not harmful but beneficial, while accepting that much higher levels of radiation are hazardous. Arguments for hormesis center around some large-scale epidemiological studies and the evidence from animal irradiation experiments, but most notably the recent advances in knowledge of the adaptive response. Proponents of radiation hormesis typically claim that radio-protective responses in cells and the immune system not only counter radiation’s harmful effects but also inhibit spontaneous cancer not related to radiation exposure.
See also: LNT Model