The term neutrino comes from Italian, meaning “little neutral one,” and neutrinos are denoted by the Greek letter ν (nu). There are three types of charged leptons, each associated with neutrino, forming three generations (between generations, particles differ by their quantum number and mass). The first generation consists of the electron (e−) and electron-neutrino (νe). The second-generation consist of the muon (μ−) and muon neutrino (νμ) The third generation consist of the tau (τ−) and the tau neutrino (ντ). Each type of neutrino is associated with an antimatter particle, called an antineutrino, which also has a neutral electric charge and 1/2 spin. Currently (2015), it is not resolved whether the neutrino and its antiparticle are not identical particles.
Carrying no electric charge, they are not affected by electromagnetic forces that act on other charged leptons, such as electrons. Since neutrinos belong to the family of leptons, they are not subject to strong force. Neutrinos are subject to the weak force, which is of a much shorter range than the electromagnetic force and gravity force. Therefore, neutrinos are the most penetrating subatomic particles, capable of passing through Earth without any interaction. It is estimated neutrinos have interaction cross-sections about 20 orders of magnitude less than typical cross-sections of scattering of two nucleons (~10-47m2 = 10-19barn). It is estimated neutrino cross-section for interaction increases linearly with the energy of the incident neutrino.
Reference: Griffiths, David, Introduction to Elementary Particles, Wiley, 1987.
See also: Antineutrino
See also: Nuclear Reactor as the Antineutrino Source.
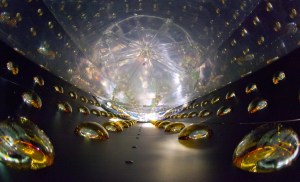
Photo: Roy Kaltschmidt, LBNL
Source: Daya Bay Reactor Neutrino Experiment
Discovery of the Neutrino
The study of beta decay provided the first physical evidence for the existence of the neutrino. The discovery of the neutrino is based on the law of conservation of energy during beta decay.
The resulting particle (alpha particle or photon) has a narrow energy distribution in both alpha and gamma decay. The particle carries the energy from the difference between the initial and final nuclear states. For example, in the case of alpha decay, when a parent nucleus breaks down spontaneously to yield a daughter nucleus and an alpha particle, the sum of the mass of the two products does not quite equal the mass of the original nucleus (see Mass Defect). As a result of the law of conservation of energy, this difference appears in the form of the kinetic energy of the alpha particle. Since the same particles appear as products at every breakdown of a particular parent nucleus, the mass difference should always be the same, and the kinetic energy of the alpha particles should also always be the same. In other words, the beam of alpha particles should be monoenergetic.
It was expected that the same considerations would hold for a parent nucleus breaking down to a daughter nucleus and a beta particle. Because only the electron and the recoiling daughter nucleus were observed beta decay, the process was initially assumed to be a two-body process, very much like alpha decay. It would seem reasonable to suppose that the beta particles would also form a monoenergetic beam.
To demonstrate the energetics of two-body beta decay, consider the beta decay in which an electron is emitted and the parent nucleus rest. Conservation of energy requires:
Since the electron is a much lighter particle, it was expected that it would carry away most of the released energy, which would have a unique value Te-.
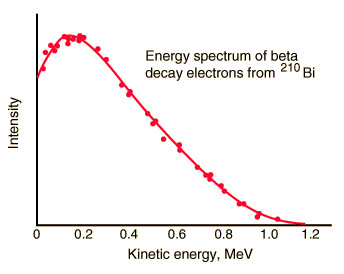
But the reality was different. However, the spectrum of beta particles measured by Lise Meitner and Otto Hahn in 1911 and by Jean Danysz in 1913 showed multiple lines on a diffuse background. Moreover, virtually all of the emitted beta particles have energies below predicted by energy conservation in two-body decays. The electrons emitted in beta decay have a continuous rather than a discrete spectrum that appeared to contradict conservation of energy, under the then-current assumption that beta decay is the simple emission of an electron from a nucleus. When this was first observed, it appeared to threaten the survival of one of the most important conservation laws in physics!
To account for this energy release, Pauli proposed (in 1931) that there was emitted in the decay process another particle, later named by Fermi the neutrino. It was clear, this particle must be highly penetrating and that the conservation of electric charge requires the neutrino to be electrically neutral. This would explain why it was so hard to detect this particle. The term neutrino comes from Italian, meaning “little neutral one,” and neutrinos are denoted by the Greek letter ν (nu). In the process of beta decay, the neutrino carries the missing energy, and also, in this process, the law of conservation of energy remains valid.
Production of Neutrinos
Neutrinos can be produced in several ways. The most powerful source of neutrinos in the solar system is doubtless the Sun itself. Billions of solar neutrinos per second pass (mostly without any interaction) through every square centimeter (~6 x 1010 cm-2s-1) on the Earth’s surface. In the Sun, neutrinos are produced after the fusion reaction of two protons during positive beta decay of helium-2 nucleus.
Each nuclear reactor is also a very powerful source of neutrinos. In fact, antineutrinos. A nuclear reactor occurs especially the β− decay because the common feature of the fission products is an excess of neutrons (see Nuclear Stability). An unstable fission fragment with the excess of neutrons undergoes β− decay, where the neutron is converted into a proton, an electron, and an electron antineutrino.
Reference: Griffiths, David, Introduction to Elementary Particles, Wiley, 1987.