Deterministic effects (or non-stochastic health effects) are health effects that are related directly to the absorbed radiation dose, and the severity of the effect increases as the dose increases. Deterministic effects have a threshold below which no detectable clinical effects do occur. The threshold may be very low (of the order of magnitude of 0.1 Gy or higher) and may vary from person to person. For doses between 0.25 Gy and 0.5 Gy, slight blood changes may be detected by medical evaluations, and for doses between 0.5 Gy and 1.5 Gy, blood changes will be noted. Symptoms of nausea, fatigue, and vomiting occur.
Once the threshold has been exceeded, the severity of an effect increases with the dose. The reason for the presence of this threshold dose is that radiation damage (serious malfunction or death) of a critical population of cells (high doses tend to kill cells) in a given tissue needs to be sustained before an injury is expressed in a clinically relevant form. Therefore, deterministic effects are also termed tissue reactions. They are also called non-stochastic effects to contrast with chance-like stochastic effects (e.g., cancer induction).
Deterministic effects are not necessarily more or less serious than stochastic effects. High doses can cause visually dramatic radiation burns and/or rapid fatality through acute radiation syndrome. Acute doses below 250 mGy are unlikely to have any observable effects. Acute doses of about 3 to 5 Gy have a 50% chance of killing a person some weeks after the exposure if a person receives no medical treatment. Deterministic effects can ultimately lead to a temporary nuisance or also to a fatality. Examples of deterministic effects:
Examples of deterministic effects are:
- Acute radiation syndrome, by acute whole-body radiation
- Radiation burns, from radiation to a particular body surface
- Radiation-induced thyroiditis, a potential side effect of radiation treatment against hyperthyroidism
- Chronic radiation syndrome from long-term radiation.
- Radiation-induced lung injury, from, for example, radiation therapy to the lungs
Lethal Doses of Radiation
The lethal dose of radiation (LD) indicates the lethal amount of radiation. The median lethal dose, LDXY, is usually used in radiation protection. For example, the dose of radiation expected to cause death to 50 % of the irradiated persons within 30 days is LD50/30. LD1 is the dose expected to cause death to 1% of the irradiated persons. Consequently, LD99 is lethal for all (99%) persons irradiated. It is also very important whether a person receives some medical treatment or not. The greater an acute radiation dose is, the greater is the possibility of it killing the individual. The LD50 is estimated to be between 3 and 5 Gy for a healthy adult.
- 2.5 Sv – Dose that kills a human with a 1% risk (LD1) if the dose is received over a very short duration.
- 5 Sv – Dose that kills a human with a 50% risk within 30 days (LD50/30) if the dose is received over a very short duration. The cause of death will be loss of bone marrow function.
- 8 Sv – Dose that kills a human with a 99% risk (LD99) if the dose is received over a very short duration. At around 10 Gy, acute inflammation of the lungs can occur and lead to death.
The lethal dose data given above apply to acute gamma doses delivered in a very short time, e.g., a few minutes. More dose is required to produce the effects listed above if the dose is received for hours or longer.
Deterministic Effects and Dose Limits
In radiation protection, dose limits are set to limit stochastic effects to an acceptable level and prevent deterministic effects completely. Note that stochastic effects arise from chance: the greater the dose, the more likely the effect. Deterministic effects normally have a threshold: above this, the severity of the effect increases with the dose. Dose limits are a fundamental component of radiation protection, and breaching these limits is against radiation regulation in most countries. Note that the dose limits described in this article apply to routine operations. They do not apply to an emergency when human life is endangered, and they do not apply in emergency exposure situations where an individual attempts to prevent a catastrophic situation.
The limits are split into two groups, the public and occupationally exposed workers. According to ICRP, occupational exposure refers to all exposure incurred by workers in the course of their work, except for
- excluded exposures and exposures from exempt activities involving radiation or exempt sources
- any medical exposure
- the normal local natural background radiation.
The following table summarizes dose limits for occupationally exposed workers and the public:
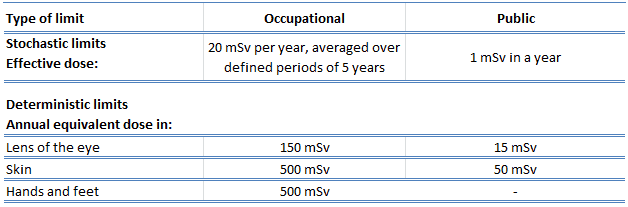
Source of data: ICRP, 2007. The 2007 Recommendations of the International Commission on Radiological Protection. ICRP Publication 103. Ann. ICRP 37 (2-4).
According to the recommendation of the ICRP in its statement on tissue reactions of 21. April 2011, the equivalent dose limit for the eye lens for occupational exposure in planned exposure situations was reduced from 150 mSv/year to 20 mSv/year, averaged over defined periods of 5 years, with no annual dose in a single year exceeding 50 mSv.
Limits on effective dose are for the sum of the relevant, effective doses from external exposure in the specified period and the committed effective dose from intakes of radionuclides in the same period. For adults, the committed effective dose is computed for 50 years after intake, whereas for children, it is computed for the period up to age 70 years. The effective whole-body dose limit of 20 mSv is an average value over five years, and the real limit is 100 mSv in 5 years, with no more than 50 mSv in any year.