A Cherenkov detector is a particle detector based on detecting Cherenkov radiation (visible light or UV photons). In contrast to a scintillation counter, the light production is instantaneous. Typical scintillator detectors have a decay time measured in microseconds, while Cherenkov radiation is nearly instantaneous and, with fast pulse processing equipment, can be measured in picoseconds. Cherenkov counters are used mainly for particle identification, i.e., to determine particle masses. Cherenkov counters contain two main elements:
- a radiator through which the charged particle passes,
- a photodetector (e.g., a photomultiplier tube – PMT).
The Cherenkov radiation, produced in the radiator, is electromagnetic radiation emitted when a charged particle (such as an electron) moves through a dielectric medium faster than the phase velocity of light in that medium. Particles exceeding the phase velocity of light result in polarization along the axis of motion creating a dipole field. When this field collapses, an electromagnetic pulse (Cherenkov radiation) is emitted in the forward direction. It is similar to the bow wave produced by a boat traveling faster than the speed of water waves. Cherenkov radiation occurs only if the particle’s speed is higher than the phase velocity of light in the material. Even at high energies, the energy lost by Cherenkov radiation is much less than that by the other mechanisms (collisions, bremsstrahlung). It is named after Soviet physicist Pavel Alekseyevich Cherenkov, who shared the Nobel Prize in physics in 1958 with Ilya Frank and Igor Tamm for the discovery of Cherenkov radiation, made in 1934.
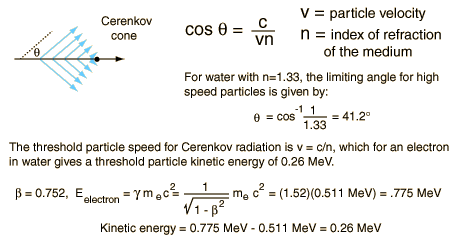
Cherenkov radiation is commonly produced through Compton electrons or pair-production electrons and positrons in dielectric materials. The intensity of light produced by this process is much less than that of luminescence (the basis for scintillation detector operation), requiring more sensitive optical photon detection equipment such as low light photomultiplier tubes (PMT). Cherenkov counters may be classified as either imaging or threshold types, depending on whether they do or do not use Cherenkov angle (θ) information. In the simple case of a threshold detector, the mass-dependent threshold energy allows the discrimination between a lighter particle (which does radiate) and a heavier particle (which does not radiate) of the same energy or momentum. Imaging counters may be used to track particles as well as identify them. Although devices using Cherenkov radiation are often thought of as particle identification (PID) detectors, in practice, they are widely used over a much broader range of applications; including:
- fast particle counters
- hadronic particle identification
- tracking detectors performing complete event reconstruction.
Types of Cherenkov Detectors – Examples
- RICH. Practical multi-track Ring-Imaging Cherenkov detectors (generically called RICH counters) are a more recent development. In a RICH detector, a cone of Cherenkov light is produced when a high-speed charged particle traverses a suitable medium, often called a radiator. This light cone is detected on a position-sensitive planar photon detector, which allows the reconstruction of a ring or disc, the radius of which is a measure for the Cherenkov emission angle. For example, the LHCb experiment on the Large Hadron Collider uses two RICH detectors for differentiating between pions and kaons.
- Super-Kamiokande. Super-Kamiokande is an underground neutrino observatory that uses large water Cherenkov counters to detect high-energy neutrinos to search for proton decay, study solar and atmospheric neutrinos, and keep watch for supernovae in the Milky Way Galaxy. It consists of a cylindrical stainless steel tank about 40 m (131 ft) in height and diameter, holding 50,000 tons of ultrapure water. Mounted on an inside superstructure are about 13,000 photomultiplier tubes that detect light from Cherenkov radiation.