Xenon Transients – Response to Reactor Power Changes
As can be seen, iodine and xenon concentrations significantly differ with neutron flux levels. Here, we will illustrate a typical xenon transient between different power levels. Recall the proportion of 135I (6.6h) and 135Xe (9.2) half-lives is very important and determines these transients, especially those with power reduction, where the xenon buildup rate is higher than xenon decay.
Consider the power increase from 50% to 100% of rated power. Consider a reactor that is operated at 50% for a long time (i.e., iodine and xenon equilibria are established). At time t0, reactor power is raised from 50% to 100% of rated power. When the reactor power increases, xenon concentration initially decreases because the burnup is increased due to a higher reaction rate. Because 95% of the xenon production is from iodine 135 decay, which has a 6.6 hours half-life, the production of xenon remains constant for several hours. After a few hours (roughly 4 to 6 hours depending on power levels), the rate of production of xenon from iodine and fission equals the rate of removal of xenon by burnup and decay. At this point, the xenon concentration reaches a minimum. The xenon concentration increases to the new equilibrium level for the new power level in roughly 40 to 50 hours. It should be noted that the magnitude and the rate of change of xenon concentration during the initial 4 to 6 hours following the power change is dependent upon the initial power level and on the amount of change in power level. The xenon concentration change is greater for a larger change in power level. For a power decrease, the behavior is reversed.
Iodine Pit – Response to Reactor Shutdown
The most spectacular and well-known phenomenon associated with xenon 135 is the behavior of a reactor after a reactor shutdown. Recall the proportion of 135I (6.6h) and 135Xe (9.2) half-lives is very important and determines these transients, especially those with power reduction, where the xenon buildup rate is higher than xenon decay.
Consider the reactor shutdown from 100% to zero. Consider the reactor that is operated at 100% for a long time (i.e., iodine and xenon equilibria are established). At time t0, reactor power fall from 100% to 0% of rated power (e.g., after SCRAM). After shutdown, xenon 135 is no longer produced by fission and is no longer removed by burnup. The only remaining production mechanism is the decay of the iodine 135, which was in the core at the time of shutdown. The only removal mechanism for xenon 135 is decay. Therefore, when the reactor power is decreased, xenon concentration initially increases because the xenon burnup falls to zero, and the 135I decay (6.6 h) is faster than the 135Xe decay (9.2 h).
The rate of the increase depends on the original neutron flux and increases with increasing flux. For large values of the neutron flux, the peak concentration occurs at 11.3 hours after shutdown (ln( λΙ/λXe)/( λI − λXe) ≈ 11.3 hours). The peak is reached when the product of the terms λΙNI is equal to λXeNXe. The amount of additional negative reactivity in the xenon peak is strongly dependent on the original neutron flux. For the reactor shutdown (LWRs) from 100% to zero, the amount of additional negative reactivity may reach up to 2500 pcm, which has very important consequences. After reaching the xenon peak, the production of xenon from iodine decay is less than the removal of xenon by decay (λΙNI < λXeNXe), and the concentration of xenon 135 decreases. After another ten half-lives (from 11.3 hours to 80 hours), all the xenon undergo beta decay. The decay of xenon 135 causes a continuous insertion of positive reactivity. This positive reactivity insertion must be considered in subcriticality maintenance (i.e., SDM) or when approaching criticality. For LWRs, the xenon 135 concentration about 20 hours after shutdown from full power will be the same as the equilibrium xenon 135 concentration at full power. About 3 days after the shutdown, the xenon 135 concentration will have decreased to a small percentage of its pre-shutdown level. The reactor can be assumed to be xenon-free without a significant error introduced into reactivity calculations.
An important consequence of this ‘xenon peak’ after a reactor shutdown is that, unless sufficient additional reactivity is present, it cannot be possible to restart the reactor again before many hours have passed. This phenomenon is known as the “iodine pit” or “xenon pit,” and it is particularly important (LWRs) near or at the end of the cycle (EOC) since there is usually insufficient positive reactivity available from the chemical shim. At the end of the cycle, the additional xenon reactivity (up to 2500 pcm) may provide sufficient negative reactivity to make the estimated critical conditions out of the allowed range because there is insufficient positive reactivity available from the control rod removal or chemical shim to counteract it. In another case, when there is sufficient reactivity to make a reactor critical, there need not be enough reactivity to increase reactor power (i.e., balance power defect).
The inability of the reactor to be started due to the effects of xenon is sometimes referred to as xenon precluded startup. It is particularly important for reactors with very small excess reactivity (e.g., Heavy Water Reactors). The period where the reactor can “override” the effects of xenon 135 is called “xenon dead time”.
Thermal power reactors are normally limited to flux levels of about 5 x 1013 neutrons/cm-2.s-1 to ensure timely restart after shutdown. For reactors with very low thermal flux levels (~5 x 1013 neutrons/cm-2.s-1 or less), most xenon is removed by decay instead of xenon burnup. Reactor shutdown does not cause any xenon 135 peaking effects for these cases. Following the peak in xenon 135 concentration about 10 hours after shutdown, the xenon 135 concentration will decrease at a rate controlled by the decay of iodine 135 into xenon 135 and the decay rate of xenon 135.
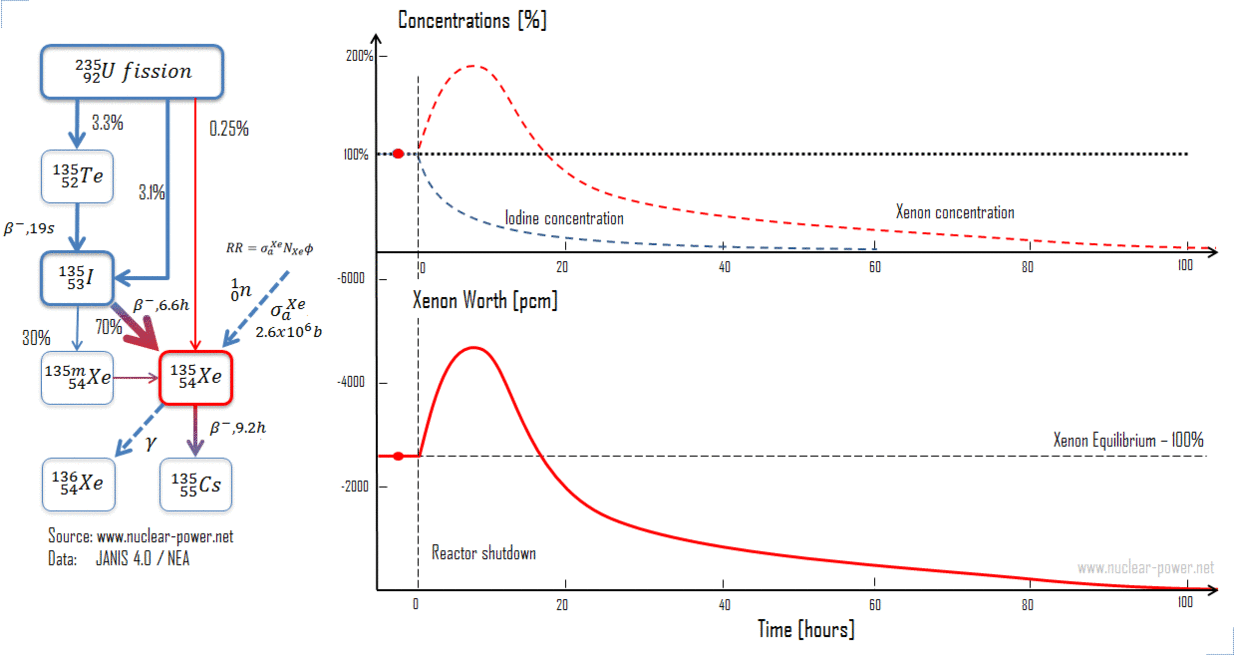