In general, nuclear fission is a nuclear reaction in which the nucleus of an atom splits into smaller parts (lighter nuclei). The fission process often produces free neutrons and photons (in the form of gamma rays) and releases a large amount of energy. Nuclear fission is either a nuclear reaction or a radioactive decay process in nuclear physics. The case decay process is called spontaneous fission, a very rare process.
Spontaneous fission is also possible if we study the nuclear binding curve, and this decay is energetically possible for a nucleus having A > 100. Although spontaneous fission is expected to become more probable as the mass number increases, it is still a rare process, even in uranium.
Spontaneous fission is feasible over practical observation times only for mass numbers greater than 232. For example, 232Th, 235U, and 238U are primordial nuclides and have left evidence of undergoing spontaneous fission in their minerals.
For heavy transuranic elements, the spontaneous fission transition rate increases with the mass number, and it may become the dominant decay mode at mass numbers greater than 260.
Similarly, as for alpha decay, spontaneous fission occurs due to quantum tunneling. Spontaneous fissions release neutrons as all fissions do, contributing to neutron flux in a subcritical reactor. Radioisotopes for which spontaneous fission is not negligible can be used as neutron sources. For example, californium-252 (half-life 2.645 years, SF branch ratio about 3.1 percent) can be used for this purpose.
The spontaneous fission of naturally occurring isotopes of uranium (uranium-238 and uranium-235) does leave trails of damage in the crystal structure of uranium-containing minerals when the fission fragments recoil through them. A radiometric dating technique based on analyses of these damage trails, or tracks, left by fission fragments in certain uranium-bearing minerals and glasses is known as fission track dating.
Spontaneous Fission of Certain Nuclei
The main isotopes, which have to be considered in the fuel cycle of all commercial light water reactors, are:
Isotopes of uranium
- 238U. 238U belongs to the group of fertile isotopes. 238U decays via alpha decay to 234Th with a half-life of ~4.5×109 years. 238U occasionally decays by spontaneous fission with a probability of 0.000055%. Its specific activity is very low ~3.4×10-7 Ci/g.
- 235U. 235U belongs to the group of fissile isotopes. 235U is the only existing fissile nucleus from naturally-occurring isotopes; therefore, it is a highly strategic material. 235U decays via alpha decay (through thorium-231) into 231Pa with a half-life of ~7×108 years. 235U occasionally decays by spontaneous fission with a very low probability of 0.0000000072%. Its specific activity is very low ~2.2×10-6 Ci/g.
- 234U. 234U belongs to the group of fertile isotopes. 234U decays via alpha decay to 230Th with a half-life of 246 000 years. 234U occasionally decays by spontaneous fission with a very low probability of 0.0000000017%. Its specific activity is much higher ~0.0063 Ci/g.
Isotopes of plutonium
- 238Pu. 238Pu belongs to the group of fertile isotopes. 238Pu decays via alpha decay to 234U with a half-life of 87.7 years. 238Pu generates very high decay heat and has a high rate of spontaneous fission.
- 239Pu. 239Pu belongs to the group of fissile isotopes, and 239Pu decays via alpha decay to 235U with a half-life of 24100 years. This isotope is the principal fissile isotope in use.
- 240Pu. 240Pu belongs to the group of fertile isotopes. 240Pu decays via alpha decay to 236U with a half-life of 6560 years. 240Pu has a very high rate of spontaneous fission and a high radiative capture cross-section for thermal and resonance neutrons.
- 241Pu. 241Pu belongs to the group of fissile isotopes. 241Pu decays via negative beta decay to 241Am with a half-life of 14.3 years. This fissile isotope decays to a non-fissile isotope with a high radiative capture cross-section for thermal neutrons. An impact on the reactivity of nuclear fuel is obvious.
Other isotopes
- 242Cm. 242Cm decays via alpha decay with a half-life of 162 days. 242Cm occasionally decays by spontaneous fission with a probability of 0.0000061%.
- 252Cf. 252Cf (half-life 2.645 years, SF branch ratio about 3.1 percent) can be used in the primary neutron source for better monitoring reactor startup and shutdown operations when the reactor is subcritical.
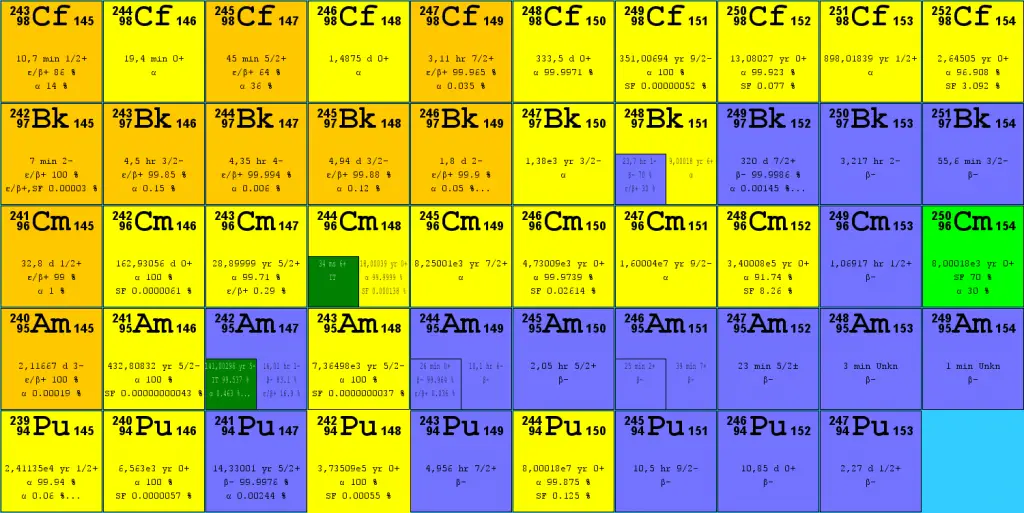
Spontaneous Fission and Source Neutrons
The term “source neutrons” refers to neutrons other than prompt or delayed fission neutrons, which come from sources other than neutron-induced fission. These neutrons are very important during reactor startup and shutdown operations when the reactor is subcritical because they allow for monitoring the subcriticality of a reactor, usually via source range excore neutron detectors.
In nuclear reactors, also spontaneous fission is very important from this point of view. In an irradiated nuclear fuel assembly, some nuclides undergo a process of spontaneous fission. As was written, spontaneous fission is, in fact, a form of radioactive decay that is found only in very heavy chemical elements (especially transuranic elements). For example, 240Pu has a very high rate of spontaneous fission. For high burnup fuel, the source neutrons are provided predominantly by the spontaneous fission of curium nuclei (Cm-242 and Cm-244).
Primary Source of Neutrons
Sometimes source neutrons must be artificially added to the system. An external source of neutrons contains a material that emits neutrons and cladding to provide a barrier between the reactor coolant and the material. External sources are usually loaded directly into the reactor core (e.g., into guide thimble tubes). Neutron sources that are based on spontaneous fission are known as primary sources. The primary source of neutrons does not need to be irradiated to produce neutrons. These sources can be used especially in the case of a first core (i.e., a core that consists of fresh fuel only). The primary source of neutrons is based on the spontaneous fission reaction. The most commonly used spontaneous fission source is the radioactive isotope californium-252. Cf-252 and all other spontaneous fission neutron sources are produced by irradiating uranium or another transuranic element in a nuclear reactor, where neutrons are absorbed in the starting material and its subsequent reaction products, transmuting the starting material into the SF isotope.