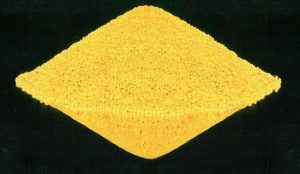
Yellowcake from Conventional Milling
After conventional milling, a fine slurry is leached in sulfuric acid (or sometimes a strong alkaline solution) to allow the separation of uranium from the waste rock. It is then recovered from the solution and precipitated as uranium oxide (U3O8) concentrate. After drying and usually heating, a yellow-brownish powder, yellowcake, is packed in 200-liter drums as a concentrate. In addition to extracting 90 to 95 percent of the uranium from the ore, the leaching agent also extracts several other “heavy metal” constituents, including molybdenum, vanadium, selenium, iron, lead, and arsenic.)
Yellowcake from ISL
In-situ leaching, also known as in-situ recovery, is a mining process different from the conventional method. It uses a chemical process to separate the uranium in the earth’s crust from the surrounding rock. The chemical solution is injected into a drilled hole into the rock at the periphery of the uranium deposit. Solutions to dissolve uranium ore are either acid (sulfuric acid or, less commonly, nitric acid) or carbonate (sodium bicarbonate). The extracted uranium solution is then filtered through resin beads, which attract uranium from the solution through an ion exchange process. Uranium-loaded resins are then transported to a processing plant, where U3O8 is separated from the resin beads, and a yellowcake is produced.
Further Processing
Before fuel fabrication, i.e., before conversion or enrichment, yellowcake is refined to obtain almost pure uranium in the form of triuranium octoxide (U3O8). Pure U3O8 is then used to prepare uranium fuel for nuclear reactors, for which triuranium octoxide powder may be sintered into fuel pellets (UO2) for use in fuel rods for pressurized heavy-water reactors. For other systems that use enriched uranium, uranium conversion and enrichment must follow this process.
Radioactivity of Yellowcake – Radioactivity of Uranium
Since yellowcake is a highly concentrated uranium powder, it must be somehow radioactive. Because the uranium in yellowcake is almost exclusively (>99%) uranium 238 with a long half-life, its specific activity is very low. 238U decays via alpha decay to 234Th with a half-life of ~4.5×109 years. 238U occasionally decays by spontaneous fission with a probability of 0.000055%. Its specific activity is very low ~3.4×10-7 Ci/g. Due to chemical leaching, yellowcake contains almost no descendants of radioactive cascades that have been in radioactive equilibrium for millions of years. These radioactive descendants were separated and disposed of in uranium tailings. Therefore, pure uranium-238 is weakly radioactive (proportional to its long half-life). Still, uranium ore is about 13 times more radioactive than the pure uranium-238 metal because of its daughter isotopes (e.g., radon, radium, etc.) it contains. Yellowcake is as radiologically harmless as natural potassium-carrying minerals or thorium-oxide mantles used in paraffin fuel lanterns.
The following figure illustrates the amount of material necessary for 37 GBq of radioactivity. Obviously, the longer the half-life, the greater the quantity of radionuclide needed to produce the same activity. Of course, the longer-lived substance will remain radioactive for much longer. As can be seen, the amount of material necessary for 37 GBq of radioactivity can vary from an amount too small to be seen (0.00088 gram of cobalt-60), through 1 gram of radium-226, to almost three tons of uranium-238.