The reaction turbine is composed of moving blades (nozzles) alternating with fixed nozzles. In the reaction turbine, the steam is expanded in fixed nozzles and also in the moving nozzles. In other words, the steam is continually expanding as it flows over the blades. There is pressure and velocity loss in the moving blades. The moving blades have a converging steam nozzle. Hence when the steam passes over the fixed blades, it expands with a decrease in steam pressure and an increase in kinetic energy.
In reaction turbines, the steam expands through the fixed nozzle, where the pressure potential energy is converted to kinetic energy. The high-velocity steam from fixed nozzles impacts the blades (nozzles), changes its direction, and undergoes further expansion. The change in its direction and the steam acceleration applies a force. The resulting impulse drives the blades forward, causing the rotor to turn. There is no net change in steam velocity across the stage but with a decrease in both pressure and temperature, reflecting the work performed in the driving of the rotor. In this type of turbine, the pressure drops occur in several stages because the pressure drop in a single stage is limited.
The main feature of this type of turbine is that the pressure drop per stage is lower in contrast to the impulse turbine, so the blades become smaller, and the number of stages increases. On the other hand, reaction turbines are usually more efficient, i.e., they have higher “isentropic turbine efficiency.” The reaction turbine was invented by Sir Charles Parsons and is known as the Parsons turbine.
In the case of steam turbines, such as would be used for electricity generation, a reaction turbine would require approximately double the number of blade rows as an impulse turbine for the same degree of thermal energy conversion. While this makes the reaction turbine much longer and heavier, the overall efficiency of a reaction turbine is slightly higher than the equivalent impulse turbine for the same thermal energy conversion.
Modern steam turbines frequently employ both reaction and impulse in the same unit, typically varying the degree of reaction and impulse from the blade root to its periphery. The rotor blades are usually designed like an impulse blade at the rot and a reaction blade at the tip.
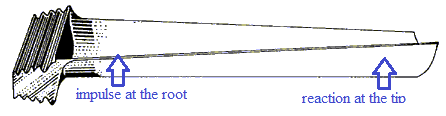
Pressure Compounding of Reaction Turbine
Compounding of steam turbines is the method in which energy from the steam is extracted in several stages rather than a single stage in a turbine. In all turbines, the rotating blade velocity is proportional to the steam velocity passing over the blade. If the steam is expanded only in a single stage from the boiler pressure to the exhaust pressure, its velocity must be extremely high.
A compounded steam turbine has multiple stages, i.e., it has more than one set of nozzles and blades, in series, keyed to the shaft or fixed to the casing, so that either the steam pressure or the jet velocity is absorbed by the turbine in several stages. For example, a large HP Turbine used in nuclear power plants can be a double-flow reaction turbine with about ten stages with shrouded blades. Large LP turbines used in nuclear power plants are usually double-flow reaction turbines with about 5-8 stages (with shrouded blades and with free-standing blades of the last three stages).
In a reaction steam turbine compounding can be achieved only in the pressure compounding. In fact, it is not exactly the same as what it was discussed in impulse turbines. Note that, there is steam expansion in both the fixed and moving blades.
References:
Reactor Physics and Thermal Hydraulics:
- J. R. Lamarsh, Introduction to Nuclear Reactor Theory, 2nd ed., Addison-Wesley, Reading, MA (1983).
- J. R. Lamarsh, A. J. Baratta, Introduction to Nuclear Engineering, 3d ed., Prentice-Hall, 2001, ISBN: 0-201-82498-1.
- W. M. Stacey, Nuclear Reactor Physics, John Wiley & Sons, 2001, ISBN: 0- 471-39127-1.
- Glasstone, Sesonske. Nuclear Reactor Engineering: Reactor Systems Engineering, Springer; 4th edition, 1994, ISBN: 978-0412985317
- Todreas Neil E., Kazimi Mujid S. Nuclear Systems Volume I: Thermal Hydraulic Fundamentals, Second Edition. CRC Press; 2 edition, 2012, ISBN: 978-0415802871
- Zohuri B., McDaniel P. Thermodynamics in Nuclear Power Plant Systems. Springer; 2015, ISBN: 978-3-319-13419-2
- Moran Michal J., Shapiro Howard N. Fundamentals of Engineering Thermodynamics, Fifth Edition, John Wiley & Sons, 2006, ISBN: 978-0-470-03037-0
- Kleinstreuer C. Modern Fluid Dynamics. Springer, 2010, ISBN 978-1-4020-8670-0.
- U.S. Department of Energy, THERMODYNAMICS, HEAT TRANSFER, AND FLUID FLOW. DOE Fundamentals Handbook, Volume 1, 2, and 3. June 1992.
- U.S. NRC. NUREG-0800, Standard Review Plan for the Review of Safety Analysis Reports for Nuclear Power Plants: LWR Edition