The supercritical water reactor (SCWR) is a concept of Generation IV reactor that is operated at supercritical pressure (i.e., greater than 22.1 MPa). The term supercritical in this context refers to the thermodynamic critical point of water (TCR = 374 °C; pCR = 22.1 MPa) and must not be confused with the criticality of the reactor core, which describes changes in the neutron population in the reactor core.
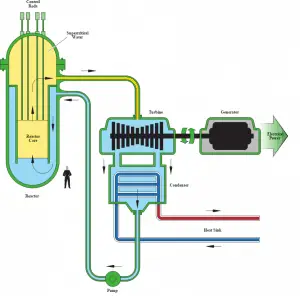
Author (Public domain): U.S. Department of Energy
Depending on the core design, the supercritical water reactor may be operated as a thermal reactor or as a fast-neutron reactor. The concept of the supercritical water reactor may be based on classical pressure vessels as in commercial PWRs or pressure tubes as in CANDU reactors. The pressure-vessel design of supercritical water reactors is developed largely in the EU, US, Japan, Korea, and China. In contrast, the pressure-channel design is developed largely in Canada and Russia. The pressure-vessel design allows using a traditional high-pressure circuit layout. The pressure-channel design allows the key features of passive accident and decay heat removal by radiation and convection from the distributed channels even with no active cooling and fuel melting. The use of multi-pass reactor flows makes reheating and superheating possible.
A once-through steam cycle has been envisaged for pressure vessel and pressure-tube designs, omitting any coolant recirculation inside the reactor. It is similar to boiling water reactors, steam will be supplied directly to the steam turbine, and the feed water from the steam cycle will be supplied back to the core.
As well as the supercritical water reactor may use light water or heavy water as a neutron moderator. As can be seen, there are many SCWR designs, but all SCWRs have a key feature, which is the use of water beyond the thermodynamic critical point as primary coolant. Since this feature increases the peak temperature, supercritical water reactors are considered a promising advancement for nuclear power plants because of their high thermal efficiency (~45 % vs. ~33 % for current LWRs).
Advantages and Challenges of SCWRs
As can be inferred from its name, the SCWR’s key feature is water use beyond the thermodynamic critical point (TCR = 374 °C; pCR = 22.1 MPa) as primary coolant. SCWR designs have unique features that offer many advantages compared to current light water reactors (LWRs).
- High thermal efficiency. Since SCWRs supply supercritical steam at pressures and temperatures much higher than in conventional LWRs, they will reach higher thermodynamic efficiency of the power plant (~45 % vs. ~33 % for current LWRs).
- Lower coolant mass flow rate. The reactor coolant flow rate of SCWR is much lower than that of BWR and PWR because the enthalpy rise in the core is much larger, which results in low capacity components of the primary system. Thus, the coolant pumps, pipes, and other supporting equipment become smaller. Moreover, the only pumps driving the coolant under normal operating conditions are the feedwater pumps and the condensate extraction pumps.
- The higher steam enthalpy allows to decrease the size of the turbine system and thus to lower the capital costs of the conventional island.
- Smaller coolant inventory. The coolant inventory is smaller, which reduces the size of the containment with pressure suppression pools.
- Since supercritical water does not undergo a phase change and exists in a single thermodynamic phase, the boiling crisis (i.e., a departure from nucleate boiling – DNB or dry out) cannot occur.
- With a direct cycle of supercritical coolant, components like steam dryers, separators, and steam generators are omitted entirely, reducing the number of major components and eliminating their associated costs.
- Many components (e.g.,, turbines) are readily developed and available from supercritical fossil-fired power plants.
The combination of advanced water-cooled reactor technology and advanced supercritical fossil technology is expected to result in a reactor concept that can be used to generate base-load electricity very economically and efficiently. This feature also makes the SCWR a very attractive concept for utilities, especially those that have experience with both water-cooled reactors and supercritical fossil plants.
On the other hand, numerous challenges must be solved before the first power plants of this type can be built:
- The cladding materials must withstand higher temperatures (600-650°C in normal operating conditions) than in today’s LWRs.
- The heat transfer phenomena from the cladding surface into supercritical fluid must be understood thoroughly, especially in the case of within transients with depressurization from supercritical to subcritical conditions.
- Due to the smaller coolant inventory, the dry out of the core progresses faster in case of a loss of coolant accident (LOCA).
Characteristics of SCWRs
SCWRs operate at pressures above the critical pressure, properties of water in the reactor change gradually and continuously from those we ordinarily associate with a liquid (high density, small compressibility) to those of a gas (low density, large compressibility) without a phase change. There is no change in the phase of water in the core. On the other hand, physical properties such as density, specific heat, specific enthalpy undergo significant changes, especially in the temperature range of the pseudocritical region (for 25 MPa between 372°C and 392°C). For example,
- the density of supercritical water at the inlet and the outlet is about 777 kg/m3 (for 25MPa and 280°C) and 90 kg/m3 (for 25MPa and 500°C),
- the specific enthalpy of supercritical water at the inlet and the outlet is about 1230 kJ/kg (for 25MPa and 280°C) and 3165 kJ/kg (for 25MPa and 500°C)
From a neutronic point of view, the density of water is the most important factor. There is a significant change in the neutron spectrum, which changes with the axial coordinate of the core. It is caused by the greater moderation in places with greater density. For this reason, a coupled neutronics – thermal-hydraulics calculation is inevitable to obtain neutron flux distribution within the core. There is a direct proportionality between the neutron flux and the reactor thermal power in each nuclear reactor.
Since the coolant (moderator) undergoes a significant change in density, the SCWR can also be designed as a fast neutron reactor. This property depends on a certain reactor design.