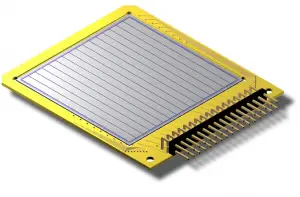
Silicon-based semiconductor detectors are mainly used for charged particle detectors (especially for tracking charged particles) and soft X-ray detectors, while germanium is widely used for gamma-ray spectroscopy. A large, clean, and almost perfect semiconductor is ideal as a counter to radioactivity. However, it isn’t easy to make large crystals with sufficient purity. The semiconductor detectors have low efficiency, but they give a very precise measure of energy. Detectors based on silicon have sufficiently low noise even at room temperature. This is caused by the large band gap of silicon (Egap= 1.12 eV), which allows us to operate the detector at room temperature, but cooling is preferred to reduce noise. The drawback is that silicon detectors are much more expensive than cloud or wire chambers and require sophisticated cooling to reduce leakage currents (noise). They also suffer degradation over time from radiation. However, this can be greatly reduced thanks to the Lazarus effect.
Principle of Operation of Silicon Detectors
The operation of semiconductor detectors is summarized in the following points:
- Ionizing radiation enters the sensitive volume of the detector and interacts with the semiconductor material.
- Particle passing through the detector ionizes the semiconductor atoms, producing the electron-hole pairs. The number of electron-hole pairs is proportional to the energy of the radiation to the semiconductor. As a result, many electrons are transferred from the valence band to the conduction band, and an equal number of holes are created in the valence band.
- Under the influence of an electric field, electrons and holes travel to the electrodes, where they result in a pulse that can be measured in an outer circuit,
- This pulse carries information about the energy of the original incident radiation. The number of such pulses per unit time also gives information about the intensity of the radiation.
The energy required to produce electron-hole pairs is very low compared to the energy required to produce paired ions in a gaseous ionization detector. In semiconductor detectors, the statistical variation of the pulse height is smaller, and the energy resolution is higher. As the electrons travel fast, the time resolution is also very good. Compared with gaseous ionization detectors, the density of a semiconductor detector is very high, and charged particles of high energy can give off their energy in a semiconductor of relatively small dimensions.
Application of Silicon Detectors
Since silicon-based detectors are very good for tracking charged particles, they constitute a substantial part of the detection system at the LHC in CERN. In principle, most silicon particle detectors work by doping narrow (usually around 100 micrometers wide) silicon strips to turn them into diodes, which are then reverse biased. As charged particles pass through these strips, they cause small ionization currents that can be detected and measured. Arranging thousands of these detectors around a collision point in a particle accelerator can accurately predict what paths particles take. For example, the Inner Tracking System (ITS) of a Large Ion Collider Experiment (ALICE) contains three layers of silicon-based detectors:
- Silicon Pixel Detector (SPD)
- Silicon Drift Detector (SDD)
- Silicon Strip Detector (SSD)
Silicon Strip Detectors
Silicon-based detectors are very good for tracking charged particles. A silicon strip detector is an arrangement of strip-like shaped implants acting as charge-collecting electrodes.
Silicon strip detectors 5 x 5 cm2 in the area are quite common and are used in series (just like planes of MWPCs) to determine charged-particle trajectories to position accuracies of the order of several μm in the transverse direction. These implants form a one-dimensional array of diodes on a low-doped, fully depleted silicon wafer. A position-sensitive detector is built by connecting each metalized strip to a charge-sensitive amplifier. Two-dimensional position measurements can be achieved by applying additional strip-like doping on the wafer backside using double-sided technology. Such devices can measure small impact parameters and thereby determine whether some charged particle originated from a primary collision or was the decay product of a primary particle that traveled a small distance from the original interaction and then decayed.
Silicon strip detectors constitute a substantial part of the detection system at the LHC in CERN. In principle, most silicon particle detectors work by doping narrow (usually around 100 micrometers wide) silicon strips to turn them into diodes, which are then reverse biased. As charged particles pass through these strips, they cause small ionization currents that can be detected and measured. Arranging thousands of these detectors around a collision point in a particle accelerator can accurately predict what paths particles take.
For example, the Inner Tracking System (ITS) of a Large Ion Collider Experiment (ALICE) contains three layers of silicon-based detectors:
- Silicon Pixel Detector (SPD)
- Silicon Drift Detector (SDD)
- Silicon Strip Detector (SSD)
Delta E – E Detector – Telescope
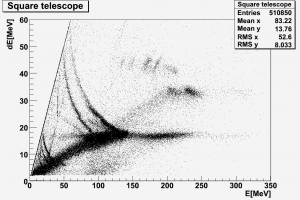
In experimental physics, ΔE-E detectors, known as telescopes, are powerful devices for charged particle identification. Telescopes consisting of pairs of thin and thick surface-barrier detectors can be used to provide charged-particle identification. These detectors must be positioned in series. The velocity is deduced from the stopping power measured in the thin detectors (ΔE detectors). There is a strong correlation between the energy deposited in each detector. This correlation depends on the mass (A), the charge (Z), and the kinetic energy (E) of each particle. The mass is deduced from the range or the total kinetic energy loss in the thicker detector (E detector).
Telescopes can be composed of several detectors (ionization chambers, silicon detectors, and scintillators, for instance) stacked to slow down incident charged particles, the first detector being the thinnest and the last the thickest. CsI scintillation counters can be, for example, used as final E counters. As an example of the telescope, an assembly based on two front ΔE silicon detectors (10 or 30 µm) and an E silicon counter 1500 µm thick may be used to detect high-energy charged particles.