Terrestrial radiation refers to radiation sources in the soil, water, and vegetation. The major isotopes of concern for terrestrial radiation are potassium, uranium, and the decay products of uranium, such as thorium, radium, and radon. Note that terrestrial radiation includes an external exposure caused by these radionuclides. An internal dose caused by these radionuclides is discussed in: Internal Source of Radiation.
These radionuclides are in trace amounts all around us. When the Earth was formed, many radioactive elements were formed. After four billion years, all the shorter-lived isotopes have decayed. But some of these isotopes have very long half-lives, billions of years, and are still present. These radionuclides are known as primordial radionuclides and contribute to the annual dose of an individual. Because most natural radioactive isotopes are heavy, more than one disintegration is necessary before reaching a stable atom. This sequence of unstable atomic nuclei and their modes of decays, which leads to a stable nucleus, is known as the radioactive series.
All-natural radionuclides are usually divided into two groups depending upon their origin:
- Primordial radionuclides. Primordial radionuclides are radionuclides found on the Earth that had existed in their current form since before Earth was formed. Primordial radionuclides are residues from the Big Bang, cosmogenic sources, and ancient supernova explosions before the solar system’s formation. Bismuth, thorium, uranium, and plutonium are primordial radionuclides because they have half-lives long enough to be still found on the Earth. Potassium-40 also belongs to primordial nuclides.
- Cosmogenic radionuclides. Cosmogenic radionuclides are those which are continually being produced by the interaction of cosmic rays.
Dose from Terrestrial Radiation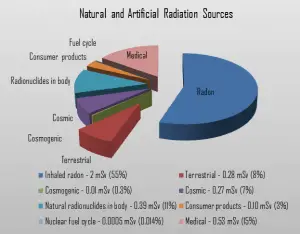
Low levels of uranium, thorium and their decay products are found everywhere. Some of these materials are ingested with food and water, while others, such as radon, are inhaled. The dose from terrestrial sources also varies in different parts of the world, and locations with higher concentrations of uranium and thorium in their soil have higher dose levels. The average dose rate from terrestrial nuclides (except radon exposure) is about 0.057 µGy/hr. The maximum values have been measured on monazite sand in Guarapari, Brazil (up to 50 µGy/hr and in Kerala, India (about 2 µGy/hr), and on rocks with a high radium concentration in Ramsar, Iran (from 1 to 10 µGy/hr).
The major isotopes of concern for terrestrial radiation are uranium and the decay products of uranium, such as thorium, radium, and radon. Radon is usually the largest natural source of radiation contributing to the exposure of public members, sometimes accounting for half the total exposure from all sources. It is so important that it is usually treated separately. The average annual radiation dose to a person from radon and its decay products is about 2 mSv/year, and it may vary over many orders of magnitude from place to place.
Radon – Health Effects
Radon is a colorless, odorless, tasteless noble gas, occurring naturally as the decay product of radium. All isotopes of radon are radioactive, but the two radon isotopes, radon-222 and radon-220, are very important from a radiation protection point of view.
- Radon-222. The radon-222 isotope is a natural decay product of the most stable uranium isotope (uranium-238); thus, it is a member of the uranium series.
- Radon-220. The radon-220 isotope, commonly referred to as thoron, is a natural decay product of the most stable thorium isotope (thorium-232). Thus it is a member of the thorium series.
It is important to note that radon is a noble gas, whereas all its decay products are metals. The main mechanism for radon entry into the atmosphere is diffusion through the soil. As a gas, radon diffuses through rocks and the soil. When radon disintegrates, the daughter metallic isotopes are ions that will be attached to other molecules like water and aerosol particles in the air. Therefore all discussions of radon concentrations in the environment refer to radon-222. While the average rate of production of radon-220 (thoron) is about the same as that of radon-222, the amount of radon-220 in the environment is much less than that of radon-222 because of the significantly shorter half-life (it has less time to diffuse) of radon-222 (55 seconds, versus 3.8 days respectively). Simply radon-220 has a lower chance of escaping from bedrock.
See also: Radon – Health Effects
Radon-222
Radon-222 is a gas produced by the decay of radium-226. Both are a part of the natural uranium series. Since uranium is found in soil throughout the world in varying concentrations, also the dose of gaseous radon varies throughout the world. Radon-222 is the most important and most stable isotope of radon, and it has a half-life of only 3.8 days, making radon one of the rarest elements since it decays away quickly. Radon gas is an important source of natural radiation, which seeps continuously from bedrock but can accumulate in poorly ventilated houses because of its high density. The fact radon is gas plays a crucial role in the spreading of all its daughter nuclei. Simply radon is a transport medium from bedrock to the atmosphere (or inside buildings) for its short-lived decay products (Pb-210 and Po-210), which posses much more health risks.
Radioactive Series in Nature
Radioactive series (also known as radioactive cascades) are three naturally occurring radioactive decay chains and one artificial radioactive decay chain of unstable heavy atomic nuclei that decay through a sequence of alpha and beta decays until a stable nucleus is achieved. Most radioisotopes do not decay directly to a stable state, and all isotopes within the series decay similarly. In the physics of nuclear decays, the disintegrating nucleus is usually referred to as the parent nucleus, and the nucleus remains as the daughter nucleus after the event. Since alpha decay represents the disintegration of a parent nucleus to a daughter through the emission of the nucleus of a helium atom (which contains four nucleons), there are only four decay series. Therefore, the mass number of the members within each series may be expressed as four times an appropriate integer (n) plus the constant for that series. As a result, the thorium series is known as the 4n series, the neptunium series as the 4n + 1 series, the uranium series as the 4n + 2 series, and the actinium series as the 4n + 3 series.
Three of the sets are called natural or classical series. The fourth set, the neptunium series, is headed by neptunium-237. Its members are produced artificially by nuclear reactions and do not occur naturally.
- the thorium series (4n series),
- the uranium series (4n+2 series),
- the actinium series (4n+3 series),
- the neptunium series (4n+1 series).
The classical series is headed by primordial unstable nuclei. Primordial nuclides are nuclides found on the Earth that had existed in their current form since before Earth was formed. The previous four series consist of the radioisotopes, which are the descendants of four heavy nuclei with long and very long half-lives:
- the thorium series with thorium-232 (with a half-life of 14.0 billion years),
- the uranium series with uranium-238 (which lives for 4.47 billion years),
- the actinium series with uranium-235 (with a half-life of 0.7 billion years).
- the neptunium series with neptunium-237 (with a half-life of 2 million years).
The half-lives of all the daughter nuclei are extremely variable, and it isn’t easy to represent a range of timescales going from individual seconds to billions of years. Since daughter radioisotopes have different half-lives, the secular equilibrium is reached after some time. In the long decay chain for a naturally radioactive element, such as uranium-238, where all of the elements in the chain are in secular equilibrium, each of the descendants has built up to an equilibrium amount, and all decay at the rate set by the original parent. If and when equilibrium is achieved, each successive daughter isotope is present in direct proportion to its half-life. Since its activity is inversely proportional to its half-life, each nuclide in the decay chain finally contributes as many individual transformations as the head of the chain.
As shown in the figures, branching occurs in all four radioactive series, meaning the decay of a given species may occur in more than one way. For example, in the thorium series, bismuth-212 decays partially by negative beta emission to polonium-212 and partially by alpha emission to thallium-206.
Radioactive cascade significantly influences radioactivity (disintegrations per second) of natural samples and natural materials. All the descendants are present, at least transiently, in any natural sample, whether metal, compound or mineral. For example, pure uranium-238 is weakly radioactive (proportional to its long half-life). Still, uranium ore is about 13 times more radioactive than the pure uranium-238 metal because of its daughter isotopes (e.g., radon, radium, etc.) it contains. Not only are unstable radium isotopes significant radioactivity emitters, but as the next stage in the decay chain, they also generate radon, a heavy, inert, naturally occurring radioactive gas. Radon is a radioactive noble gas, but the main issue is that it is a transport medium from bedrock to the atmosphere (or inside buildings), for its short-lived decay products (Pb-210 and Po-210) posses much more health risks.
Radiation from Uranium and its Decay Products
Uranium cascade significantly influences radioactivity (disintegrations per second) of natural samples and natural materials. All the descendants are present, at least transiently, in any natural uranium-containing sample, whether metal, compound or mineral. For example, pure uranium-238 is weakly radioactive (proportional to its long half-life). Still, uranium ore is about 13 times more radioactive than the pure uranium-238 metal because of its daughter isotopes (e.g., radon, radium, etc.) it contains. Not only are unstable radium isotopes significant radioactivity emitters, but as the next stage in the decay chain, they also generate radon, a heavy, inert, naturally occurring radioactive gas.
Radiation from Thorium and its Decay Products
Thorium cascade significantly influences radioactivity(disintegrations per second) of natural samples and natural materials. All the descendants are present, at least transiently, in any natural thorium-containing sample, whether metal, compound or mineral. For example, pure thorium-232 is weakly radioactive (proportional to its long half-life). Still, a thorium ore is about 10 times more radioactive than the pure thorium-232 metal because of its daughter isotopes (e.g., radon, radium, etc.) it contains. Not only are unstable radium isotopes significant radioactivity emitters, but as the next stage in the decay chain, they also generate radon, a heavy, inert, naturally occurring radioactive gas.
Liquid Earth’s Core
All three naturally-occurring isotopes of uranium (238U, 235U, and 234U) and naturally-occurring isotope of thorium have a very long half-life (e.g., 4.47×109 years for 238U). Because of this long half-life, uranium and thorium are weakly radioactive and contribute to low levels of natural background radiation in the environment. These isotopes are alpha radioactive (emitting alpha particles), but they can also rarely undergo spontaneous fission.
All naturally-occurring isotopes belong to primordial nuclides because their half-life is comparable to the age of the Earth (~4.54×109 years). Uranium has the second-highest atomic mass of these primordial nuclides, lighter only than plutonium. Moreover, the decay heat of uranium and thorium and their decay products (e.g., radon, radium, etc.) contribute to heating the Earth’s core. Together with potassium-40 in the Earth’s mantle, these elements are the main source of heat that keeps the Earth’s core liquid.
Terrestrial Radiation – Is it dangerous?
We must emphasize that eating bananas, working as an airline flight crew, or living in locations, increases your annual dose rate. But it does not mean that it must be dangerous. In each case, the intensity of radiation also matters. It is very similar to heat from a fire (less energetic radiation). If you are too close, the intensity of heat radiation is high, and you can get burned. If you are at the right distance, you can withstand it without any problems, and it is comfortable. If you are too far from a heat source, the insufficiency of heat can also hurt you. In a certain sense, this analogy can be applied to radiation also from radiation sources.
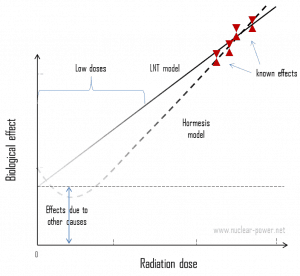
In the case of terrestrial radiation, we usually talk about so-called “low doses.” A low dose means additional small doses comparable to the normal background radiation (10 µSv = average daily dose received from natural background). The doses are very low; therefore, cancer induction’s probability could be almost negligible. Secondly, and this is crucial, the truth about low-dose radiation health effects still needs to be found. It is unknown whether these low doses of radiation are detrimental or beneficial (and where is the threshold). Government and regulatory bodies assume an LNT model instead of a threshold or hormesis not because it is the more scientifically convincing but because it is, the more conservative estimate. The problem with this model is that it neglects many defense biological processes that may be crucial at low doses. The research during the last two decades is very interesting and shows that small doses of radiation given at a low dose rate stimulate the defense mechanisms. Therefore the LNT model is not universally accepted, with some proposing an adaptive dose-response relationship where low doses are protective, and high doses are detrimental. Many studies have contradicted the LNT model, and many have shown adaptive response to low dose radiation resulting in reduced mutations and cancers. This phenomenon is known as radiation hormesis.