In general, many instabilities may occur in two-phase systems. In nuclear engineering, study of the multiphase flow stability is of importance in accident management of pressurized water reactors and of the highest importance in normal/abnormal conditions in boiling water reactors.
In PWRs at normal operation, the flow is considered to be single-phase. But a great deal of study has been performed on the flow instability in case of transients and accidents (such as the loss-of-coolant accident – LOCA or trip of RCPs with presence of natural circulation), in which flow oscillations or flow reversals may occur.
Flow oscillations are variations in flow caused by void formations, which are undesirable for several reasons.
- Flow oscillations can cause undesirable mechanical stress on fuel components (such as spacing grids). This can lead to the failure of those components due to fatigue.
- Flow oscillations affect the local heat transfer characteristics. In the case of PWRs, the critical safety issue is named DNB (departure from nucleate boiling), which causes the formation of a local vapor layer, causing a dramatic reduction in heat transfer capability. It has been found through testing that the critical heat flux (CHF) required for departure from nucleate boiling (DNB) can be lowered by as much as 40% when the flow is oscillating. This severely reduces the thermal limit and the power density along the length of the reactor core.
Flow oscillations can be a problem during natural circulation operations (e.g.,, after tripping of RCPs). Natural circulation is an important design feature and ultimate heat removal mechanism. Because of the low flow rates present, coolant boiling may occur, which may form flow oscillations. During natural circulation, the steam bubbles formed during a flow oscillation may have enough effect to cause complete flow reversal in the affected channel.
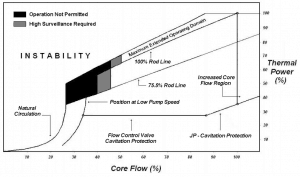
In BWRs, evaporation of coolant occurs at normal operation, and it is a very desired phenomenon. On the other hand, convective evaporation in the fuel channel causes that the flow pattern changes along the fuel channel depending on the flow rate and thermal power. It has been found that there are instability regions in which two-phase flow instabilities may arise. These two-phase flow instabilities are undesirable as they can result in mechanical vibrations and system control problems, affect normal operation, restrict operating parameters, and influence reactor safety. It must be noted flow stability in BWRs is not a major issue for many years because it is a well-known phenomenon.
In general, there are many classifications of flow instabilities. The following classification is based upon thermal-hydraulic fundamental mechanisms:
The static instabilities are:
- Flow excursion
- Boiling crisis
- Relaxation types, including flow pattern transition
The dynamic instabilities are:
- Density wave oscillations
- Pressure drop oscillations
- Thermal oscillations.
The proper characterization of the instabilities and the condition for their occurrence can determine the optimal and safe operation of the systems. The most accepted explanation for the dynamic type of instabilities is called density wave oscillations (DWO).
The density wave causes a delay in the local pressure drop caused by a change in inlet flow. Because of this delay, the sum of all local pressure drops may result in a total drop that is out-of-phase with the inlet flow. The basic mechanism causing flow instabilities in BWRs is the density wave. The characteristic periods of these oscillations are associated with the time required for a fluid particle to travel through the entire loop.
Types of instabilities observed in BWRs
- Control System Instabilities. Control system instabilities are related to the action of controllers that, through actuators, attempt to regulate some of the variables of the reactor.
- Channel Flow Instabilities. This type of instability can be described as follows: Let assume a flow perturbation. This perturbation causes a “wave” of voids traveling upward through the channel, producing a two-phase pressure drop (pressure drop increases significantly as void fraction increases) that is delayed with respect to the original perturbation. An increase in channel pressure drop (density wave) may lead to instability in the flow rate.
- Coupled Neutronic-Thermohydraulic Instability. The dominant type of instabilities in commercial BWRs is the coupled neutronic-thermohydraulic instability (also known as reactivity instability). The power generation in BWRs is directly related to the fuel neutron flux, which is strongly related to the average void fraction in the core channels through. This effect is known as reactivity feedback. The reactivity feedback caused by changes in void fraction (void coefficient) is delayed as the voids travel upward through the fuel channel. In some cases, the delay may be long enough, and the void feedback may be strong enough that the reactor configuration becomes unstable. In this case, the neutron flux may oscillate.
Special References:
- Francesco D’Auria, The BWR Stability Issue, THICKET 2008 – Session IX – Paper 26
- Dag Strømsvåg, Fundamental mechanisms of density wave oscillations and the effect of subcooling, NTNU, 2011.
- J. March-Leuba, Density -Wave Instabilities in Boiling Water Reactors. NUREG/CR-6003, ORNL, 1992.