As for another differential equation, the solution is given by boundary and initial conditions. Several common possibilities are simply expressed in the mathematical form concerning the boundary conditions.
Because the heat equation is second order in the spatial coordinates, two boundary conditions must be given for each direction of the coordinate system. Heat transfer is significant to describe a heat transfer problem completely. Therefore, we need to specify four boundary conditions for two-dimensional problems and six boundary conditions for three-dimensional problems.
Four kinds of boundary conditions commonly encountered in heat transfer are summarized in following section:
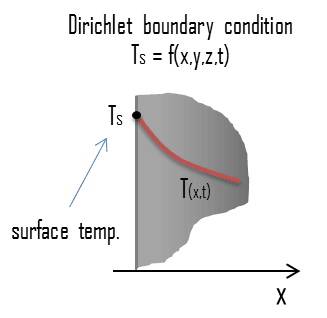
This condition corresponds to a given fixed surface temperature in heat transfer problems. The Dirichlet boundary condition is closely approximated, for example, when the surface is in contact with a melting solid or a boiling liquid. In both cases, there is heat transfer at the surface, while the surface remains at the temperature of the phase change process.
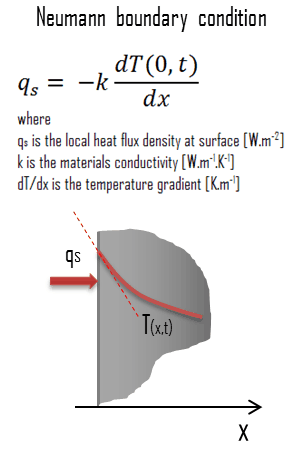
In heat transfer problems, the Neumann condition corresponds to a given rate of change of temperature. In other words, this condition assumes that the heat flux at the material’s surface is known. The heat flux in the positive x-direction anywhere in the medium, including the boundaries, can be expressed by Fourier’s law of heat conduction.
Special Case – Adiabatic Boundary – Perfectly Insulated Boundary
A special case of this condition corresponds to the perfectly insulated surface for which (∂T/∂x = 0). Heat transfer through a properly insulated surface can be taken to be zero since adequate insulation reduces heat transfer through a surface to negligible levels. Mathematically, this boundary condition can be expressed as:
Special Case – Thermal Symmetry
Another very important case that can be used for solving heat transfer problems involving fuel rods is thermal symmetry. For example, the two surfaces of a large hot plate of thickness L suspended vertically in the air will be subjected to the same thermal conditions. Thus, the temperature distribution will be symmetrical (i.e., one half of the plate will be the same temperature profile as the other half). As a result, there must be a maximum in the centerline of the plate, and the centerline can be viewed as an insulated surface (∂T/∂x = 0). The thermal condition at this plane of symmetry can be expressed as:
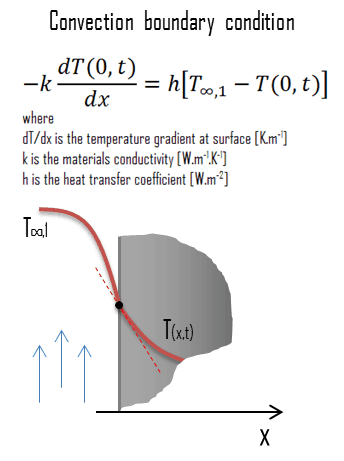
In other words, this condition assumes that the heat conduction at the material’s surface is equal to the heat convection at the surface in the same direction. Since the boundary cannot store energy, the net heat entering the surface from the convective side must leave the surface from the conduction side.
Similarly, the radiation boundary condition can be constructed and used.
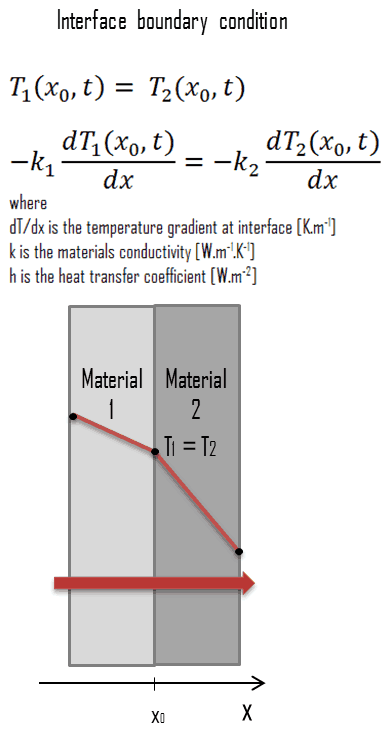
- two bodies in contact must have the same temperature at the area of contact (i.e., an ideal contact without contact resistance)
- an interface cannot store any energy, and therefore the heat conduction at the surface of the first material is equal to the heat conduction at the surface of the second material
Noteworthy, when components are bolted or otherwise pressed together, a knowledge of the thermal performance of such joints is also needed. The temperature drop across the interface between materials may be appreciable in these composite systems. This temperature drop is characterized by the thermal contact conductance coefficient, hc, which indicates the thermal conductivity, or ability to conduct heat, between two bodies in contact.
The interface boundary condition can be mathematically expressed in the way depicted in the figure.
where the function f(x, y, z) represents the temperature field inside the material at time t = 0. Note that under steady conditions, the heat conduction equation does not involve any time derivatives (∂T/∂t = 0), and thus we do not need to specify an initial condition.
- Fundamentals of Heat and Mass Transfer, 7th Edition. Theodore L. Bergman, Adrienne S. Lavine, Frank P. Incropera. John Wiley & Sons, Incorporated, 2011. ISBN: 9781118137253.
- Heat and Mass Transfer. Yunus A. Cengel. McGraw-Hill Education, 2011. ISBN: 9780071077866.
- Fundamentals of Heat and Mass Transfer. C. P. Kothandaraman. New Age International, 2006, ISBN: 9788122417722.
- U.S. Department of Energy, Thermodynamics, Heat Transfer and Fluid Flow. DOE Fundamentals Handbook, Volume 2 of 3. May 2016.
Nuclear and Reactor Physics:
- J. R. Lamarsh, Introduction to Nuclear Reactor Theory, 2nd ed., Addison-Wesley, Reading, MA (1983).
- J. R. Lamarsh, A. J. Baratta, Introduction to Nuclear Engineering, 3d ed., Prentice-Hall, 2001, ISBN: 0-201-82498-1.
- W. M. Stacey, Nuclear Reactor Physics, John Wiley & Sons, 2001, ISBN: 0- 471-39127-1.
- Glasstone, Sesonske. Nuclear Reactor Engineering: Reactor Systems Engineering, Springer; 4th edition, 1994, ISBN: 978-0412985317
- W.S.C. Williams. Nuclear and Particle Physics. Clarendon Press; 1 edition, 1991, ISBN: 978-0198520467
- G.R.Keepin. Physics of Nuclear Kinetics. Addison-Wesley Pub. Co; 1st edition, 1965
- Robert Reed Burn, Introduction to Nuclear Reactor Operation, 1988.
- U.S. Department of Energy, Nuclear Physics and Reactor Theory. DOE Fundamentals Handbook, Volume 1 and 2. January 1993.
- Paul Reuss, Neutron Physics. EDP Sciences, 2008. ISBN: 978-2759800414.
Advanced Reactor Physics:
- K. O. Ott, W. A. Bezella, Introductory Nuclear Reactor Statics, American Nuclear Society, Revised edition (1989), 1989, ISBN: 0-894-48033-2.
- K. O. Ott, R. J. Neuhold, Introductory Nuclear Reactor Dynamics, American Nuclear Society, 1985, ISBN: 0-894-48029-4.
- D. L. Hetrick, Dynamics of Nuclear Reactors, American Nuclear Society, 1993, ISBN: 0-894-48453-2.
- E. E. Lewis, W. F. Miller, Computational Methods of Neutron Transport, American Nuclear Society, 1993, ISBN: 0-894-48452-4.