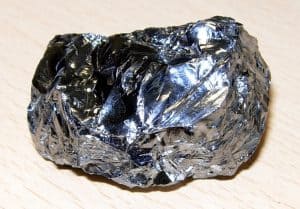
In general, semiconductors are inorganic or organic materials that can control their conduction depending on chemical structure, temperature, illumination, and the presence of dopants. The name semiconductor comes from the fact that these materials have electrical conductivity between a metal, like copper, gold, etc., and an insulator, like glass. They have an energy gap of less than 4eV (about 1eV). In solid-state physics, this energy gap or band gap is an energy range between the valence band and conduction band where electron states are forbidden. In contrast to conductors, semiconductors’ electrons must obtain energy (e.g., from ionizing radiation) to cross the band gap and reach the conduction band. Properties of semiconductors are determined by the energy gap between valence and conduction bands.
Intrinsic Semiconductor – Pure Semiconductor
An intrinsic semiconductor is a completely pure semiconductor without any significant dopant species present. Therefore, intrinsic semiconductors are also known as pure semiconductors or i-type semiconductors.
The number of charge carriers at a certain temperature is therefore determined by the material’s properties instead of the number of impurities. Note that a 1 cm3 sample of pure germanium at 20 °C contains about 4.2×1022 atoms but also contains about 2.5 x 1013 free electrons and 2.5 x 1013 holes. These charge carriers are produced by thermal excitation. In intrinsic semiconductors, the number of excited electrons and the number of holes are equal: n = p. Electrons and holes are created by the excitation of electrons from the valence band to the conduction band. An electron-hole (often simply called a hole) is the lack of an electron at a position where one could exist in an atom or atomic lattice. This equality may even be the case after doping the semiconductor, though only if it is doped with both donors and acceptors equally. In this case, n = p still holds, and the semiconductor remains intrinsic, though doped.
Semiconductors have an energy gap of less than 4eV (about 1eV). Band gaps are naturally different for different materials. For example, diamond is a wide-bandgap semiconductor (Egap= 5.47 eV) with high potential as an electronic device material in many devices. On the other side, germanium has a small band gap energy (Egap = 0.67 eV), which requires operating the detector at cryogenic temperatures. In solid-state physics, this energy gap or band gap is an energy range between the valence band and conduction band where electron states are forbidden. In contrast to conductors, semiconductors’ electrons must obtain energy (e.g., from ionizing radiation) to cross the band gap and reach the conduction band.
Intrinsic semiconductors, however, are not very useful, as they are neither very good insulators nor very good conductors. However, one important feature of semiconductors is that their conductivity can be increased and controlled by doping with impurities and gating with electric fields. Recall that a 1 cm3 sample of pure germanium at 20 °C contains about 4.2×1022 atoms but also about 2.5 x 1013 free electrons and 2.5 x 1013 holes constantly generated from thermal energy. Total absorption of a 1 MeV photon produces around 3 x 105 electron-hole pairs. This value is minor compared to the total number of free carriers in a 1 cm3 intrinsic semiconductor. As can be seen, the signal-to-noise ratio (S/N) would be minimal. Adding 0.001% of arsenic (an impurity) donates an extra 1017 free electrons in the same volume, and the electrical conductivity is increased by 10,000. The signal-to-noise ratio (S/N) in doped material would be even smaller. Because germanium has a relatively low band gap, these detectors must be cooled to reduce the thermal generation of charge carriers to an acceptable level. Otherwise, leakage current-induced noise destroys the energy resolution of the detector. Doping and gating move the conduction or valence band much closer to the Fermi level and greatly increase the number of partially filled states.