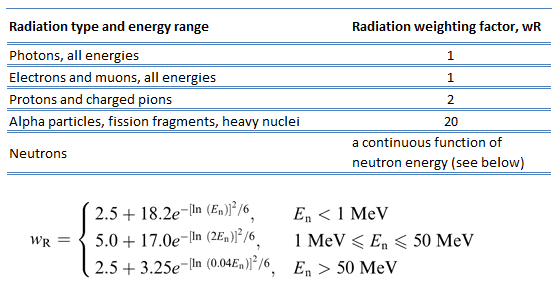
As was written, each type of radiation interacts with matter in a different way. For example, charged particles with high energies can directly ionize atoms. Alpha particles are fairly massive and carry a double positive charge, so they tend to travel only a short distance and do not penetrate very far into a tissue, if at all. However, alpha particles will deposit their energy over a smaller volume (possibly only a few cells if they enter a body) and cause more damage to those few cells.
Beta particles (electrons) are much smaller than alpha particles and carry a single negative charge. They are more penetrating than alpha particles and can travel several meters but deposit less energy at any point along their paths than alpha particles. This means beta particles tend to damage more cells but with lesser damage. On the other hand, electrically neutral particles interact indirectly but can also transfer some or all of their energies to the matter.
It would certainly simplify matters if the biological effects of radiation were directly proportional to the absorbed dose. Unfortunately, biological effects also depend on how the absorbed dose is distributed along the radiation path. Studies have shown that alpha and neutron radiation cause greater biological damage for a given energy deposition per kg of tissue than gamma radiation does. Biological effects of any radiation increase with the linear energy transfer (LET) were discovered. In short, the biological damage from high-LET radiation (alpha particles, protons, or neutrons) is much greater than that from low-LET radiation (gamma rays). This is because the living tissue can more easily repair damage from radiation spread over a large area than that concentrated in a small area. Of course, at very high levels of exposure, gamma rays can still cause a great deal of damage to tissues.
Because more biological damage is caused for the same physical dose (i.e., the same energy deposited per unit mass of tissue), one gray of alpha or neutron radiation is more harmful than one gray of gamma radiation. The fact that radiations of different types (and energies) give different biological effects for the same absorbed dose is described in terms of factors known as the relative biological effectiveness (RBE) and the radiation weighting factor (wR).
The radiation weighting factor is a dimensionless factor used to determine the equivalent dose from the absorbed dose averaged over a tissue or organ based on the type of radiation absorbed. The resulting weighted dose was designated as the organ- or tissue equivalent dose:
An equivalent dose of one Sievert represents that quantity of radiation dose that is equivalent to specified biological damage to one gray of X-rays or gamma rays. An equivalent dose is a non-physical quantity (wR is derived from biological consequences of ionizing radiation) widely used in dosimetry measured by dosimeters. The ICRP designates an equivalent dose as a “limiting quantity”; to specify exposure limits to ensure that “the occurrence of stochastic health effects is kept below unacceptable levels and those tissue reactions are avoided.”