In the 20th century, Einstein’s principle of equivalence put all observers, moving or accelerating, on the same footing. Einstein summarised this notion in a postulate:
Principle of Equivalence:
An observer cannot determine, in any way whatsoever, whether the laboratory he occupies is in a uniform gravitational field or is in a reference frame that is accelerating relative to an inertial frame.
This led to an ambiguity as to what exactly is meant by the force of gravity and weight. A scale in an accelerating elevator cannot be distinguished from a scale in a gravitational field. Gravitational force and weight thereby became essentially frame-dependent quantities. According to the general theory of relativity, gravitational and inertial mass are not different properties of matter but two aspects of a fundamental and single property of matter.
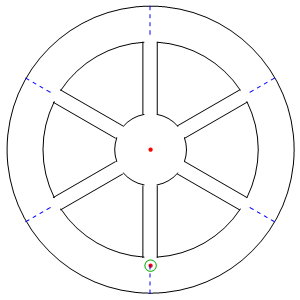
In situations in which gravitation is absent, but the chosen coordinate system is not inertial. Still, it is accelerated with the observer, then g-forces and corresponding proper accelerations felt by observers in these coordinate systems are caused by the mechanical forces which resist their weight in such systems. The most realistic method of producing artificial gravity, for example, aboard a space station, can be imitated in a rotating spaceship. Objects inside would be pushed toward the hull, and they will have some weight. This weight is produced by fictitious forces or “inertial forces,” which appear in all such accelerated coordinate systems. Unlike real gravity, which pulls towards the center of the planet, the centripetal force pushes towards the axis of rotation.