A typical thermal reactor contains about 100 tons of uranium with an average enrichment of 2% (do not confuse it with the enrichment of the fresh fuel, that is about 4%). For the reactor of power of 3000MWth, determine the consumption of 235U that must undergo fission each day to provide this thermal power.
Solution:
This problem can be solved very simply. The average recoverable energy per 235U fission is about Er = 200.7 MeV/fission. Since we know that each second we need 3000 MJ of energy, the required reaction rate can be determined directly as:
Since each atom of 235U has a mass of 235u x 1.66 x 10-27 kg/u = 3.9 x 10-25 kg, the daily consumption of a reactor is:
9.33 x 1019 fissions/sec x 3.9 x 10-25 kg x 86400 sec/day = 3.14 kg/day
For comparison, a 1000 MWe coal-fired power plant burns about 10 000 tons (about 10 million kg) of coal per day.
Since a typical fuel cycle takes about 320 days (12-month fuel cycle), the annual fuel consumption is about:
3.14 kg/day x 320 days = 1 005 kg of 235U
Typically, when uranium 235 nucleus undergoes fission, the nucleus splits into two smaller nuclei (triple fission can also rarely occur), along with a few neutrons (the average is 2.43 neutrons per fission by thermal neutron) and release of energy in the form of heat and gamma rays. The average of the fragment atomic mass is about 118, but very few fragments near that average are found. It is much more probable to break up into unequal fragments, and the most probable fragment masses are around mass 95 (Krypton) and 137 (Barium). Most fission fragments are highly unstable(radioactive) nuclei and undergo further radioactive decays to stabilize themselves.
Energy Release per Fission
In general, nuclear fission results in the release of enormous quantities of energy. The amount of energy depends strongly on the nucleus to be fissioned and also depends strongly on the kinetic energy of an incident neutron. It is necessary to identify the individual components of this energy precisely to calculate the power of a reactor. At first, it is important to distinguish between the total energy released and the energy that can be recovered in a reactor.
The total energy released in fission can be calculated from binding energies of the initial target nucleus to be fissioned and binding energies of fission products. But not all the total energy can be recovered in a reactor. For example, about 10 MeV is released in the form of neutrinos (in fact, antineutrinos). Since the neutrinos are weakly interacting (with an extremely low cross-section of any interaction), they do not contribute to the energy that can be recovered in a reactor.
See also: Energy Release from Fission
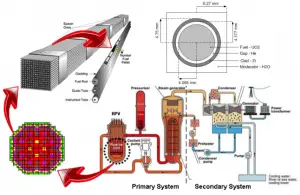
Summary:
Consumption of a 3000MWth (~1000MWe) reactor (12-months fuel cycle)
It is an illustrative example, following data do not correspond to any reactor design.
- Typical reactor may contain about 165 tonnes of fuel (including structural material)
- Typical reactor may contain about 100 tonnes of enriched uranium (i.e., about 113 tonnes of uranium dioxide).
- This fuel is loaded within, for example, 157 fuel assemblies composed of over 45,000 fuel rods.
- A common fuel assembly contain energy for approximately 4 years of operation at full power.
- Therefore about one-quarter of the core is yearly removed to the spent fuel pool (i.e., about 40 fuel assemblies). At the same time, the remainder is rearranged to a location in the core better suited to its remaining level of enrichment (see Power Distribution).
- The removed fuel (spent nuclear fuel) still contains about 96% of reusable material (it must be removed due to decreasing kinf of an assembly).
- The annual natural uranium consumption of this reactor is about 250 tonnes of natural uranium (to produce about 25 tonnes of enriched uranium).
- Annual enriched uranium consumption of this reactor is about 25 tonnes of enriched uranium.
- Annual fissile material consumption of this reactor is about 1 005 kg.
- Annual matter consumption of this reactor is about 1.051 kg.
- But it corresponds to about 3 200 000 tons of coal burned in coal-fired power plant per year.