
Spent nuclear fuel, also called the used nuclear fuel, is a nuclear fuel that has been irradiated in a nuclear reactor (usually at a nuclear power plant or an experimental reactor), and a fresh fuel must replace that due to its insufficient reactivity. Spent nuclear fuel is characterized by fuel burnup, a measure of how much energy is extracted from nuclear fuel, and a measure of fuel depletion. Due to fuel depletion and fission fragments buildup, spent nuclear fuel is no longer useful in sustaining a nuclear reaction in an ordinary thermal reactor and must be replaced by fresh fuel. It may have considerably different isotopic constituents depending on its point along the nuclear fuel cycle.
Spent Nuclear Fuel – Composition
As a reactor is operated at significant power, fuel atoms are constantly consumed, resulting in the slow depletion of the fuel. It must be noted there are also research reactors, which have very low power, and the fuel in these reactors does not change its isotopic composition.
Research reactors with significant thermal power and all power reactors are subjected to significant isotopic changes. The study of these isotopic changes is known as long-term kinetics, which describes phenomena that occur over months or even years. Most common reactor fuels are composed of either natural or partially enriched uranium. Typically, PWRs use an enriched uranium fuel (~4% of U-235) as a fresh fuel. Exposure to neutron flux gradually depletes the uranium-235, decreasing core reactivity (compensated by control rods, chemical shim, or burnable absorbers). The initial fuel load of a new reactor core (so-called first core) is entirely fresh fuel, fuel with no plutonium or fission products present. The contribution of uranium-238 directly to fission is quite small in most thermal reactors. On the other hand, uranium-238 plays a very important role since plutonium-239 is formed in a nuclear reactor from fertile isotope 238U.
Evolution Equations
The exact evolution of isotopic changes is usually modeled mathematically by a set of differential equations known as evolution equations. These equations describe the rate of burnup of U-235, the buildup of Pu-239, the production of Pu-240 and Pu-241, the buildup of neutron-absorbing fission products, and the overall rate of reactivity change in the reactor due to the changing composition of the fuel. The evolution equation can be constructed for each isotope. For example:
Special reference: W. M. Stacey, Nuclear Reactor Physics, John Wiley & Sons, 2001, ISBN: 0- 471-39127-1.
Special reference: Paul Reuss, Neutron Physics, EDP Sciences, 2008, ISBN: 2759800415.
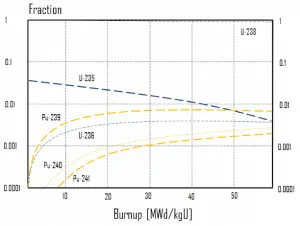
In summary, the following points are the main isotopic changes for fuel at fuel burnup of 40 GWd/tU:
- Approximately 3 – 4% of the heavy nuclei are fissioned.
- About two-thirds of these fissions come directly from uranium 235, and the other third from plutonium, which is produced from uranium 238. The contribution significantly increases as the fuel burnup increases.
- The removed fuel (spent nuclear fuel) still contains about 96% of reusable material. It must be removed due to decreasing kinf of an assembly, or in other words, it must be removed due to accumulation of fission products with significant absorption cross-section.
- Discharged fuel contains about 0.8% plutonium and about 1% uranium 235. It must be noted that there is a significant content (about 0.5%) of uranium 236, which is neither a fissile isotope nor a fertile isotope.
Fission Products in SNF
Nuclear fission products are the fragments left after a nucleus fissions. Typically, when the uranium 235 nucleus undergoes fission, the nucleus splits into two smaller nuclei, along with a few neutrons, and releases energy in the form of heat (kinetic energy of these fission fragments) and gamma rays. The average fragment mass is about 118, but very few fragments near that average are found. It is much more probable to break up into unequal fragments, and the most probable fragment masses are around mass 95 (Krypton) and 137 (Barium). The fission products include every element from zinc through to the lanthanides; much of the fission yield is concentrated in two peaks, one in the second transition row (Zr, Mo, Tc, Ru, Rh, Pd, Ag) and the other later in the periodic table (I, Xe, Cs, Ba, La, Ce, Nd). Most of these fission fragments are highly unstable (radioactive) and undergo further radioactive decays to stabilize themselves. Many of the fission products are only short-lived radioisotopes. But many are medium to long-lived radioisotopes such as 90Sr, 137Cs, 99Tc, and 129I.
An isotope that is long-lived or even stable is known as reactor slag. The accumulation of these parasitic absorbers is known as reactor slagging and determines the lifetime of nuclear fuel in a reactor since their buildup causes (together with a decrease in fissile material) a continuous decrease in core reactivity.
Samarium 149 belongs to this group of isotopes, but its importance is so high that it is usually discussed separately. As can be seen from the table, there are numerous other fission products that, as a result of their concentration and thermal neutron absorption cross-section, have a poisoning effect on reactor operation. Other fission products with relatively high absorption cross sections include 83Kr, 95Mo, 143Nd, and 147Pm. Individually, they are of little consequence but taken together and have a significant effect. These are often characterized as lumped fission product poisons.