Article Summary & FAQs
What is a nuclear reactor?
In general, a nuclear reactor is a device used to initiate and control a self-sustained nuclear chain reaction. Since nuclear reactors are used in nuclear power plants, research facilities, or nuclear-propelled ships, their output is thermal energy, or they can be used as a source of neutron radiation.
Key Facts
- The key component of nuclear reactors is a reactor core. The reactor core is a bounded region, where a neutron multiplication occurs and where chain reactions occur. The reactor core contains the nuclear fuel (fuel assemblies), the moderator, and the control rods.
- The main product of research reactors is usually a neutron flux, which is then used, for example, for medical purposes or research purposes.
- Nuclear reactors in nuclear power plants are “only” used to generate large amounts of heat. This heat is used to generate steam which drives a steam turbine connected to a generator that produces electricity.
- The most common nuclear reactors are light water reactors (LWR), where light water is used as a moderator.
- The key components common to most PWR types of nuclear reactors are:
- Nuclear reactor core.
- Reactor pressure vessel.
- Lower support structure.
- Surveillance capsule assembly.
- Core support barrel.
- Upper guide structure assembly.
- A typical reactor may contain about 100 tonnes of enriched uranium (i.e., about 113 tonnes of uranium dioxide).
- Typical reactor nominal thermal power is about 3400MW, thus corresponds to the net electric output of 1100MW. Therefore the typical thermal efficiency of its Rankine cycle is about 33%.
- In nuclear reactors, control rods are used for maintaining the desired state of fission reactions. The basic classification of states of a reactor is according to the multiplication factor. Suppose the multiplication factor for a multiplying system is equal to 1.0. In that case, there is no change in neutron population in time (i.e., reactor is stable), and the chain reaction will be self-sustaining. This condition is known as the critical state.
Definition
In general, a nuclear reactor is a device used to initiate and control a self-sustained nuclear chain reaction. Since nuclear reactors are used in nuclear power plants, research facilities, or nuclear-propelled ships, their output is thermal energy, or they can be used as a source of neutron radiation.
Classification of Nuclear Reactors
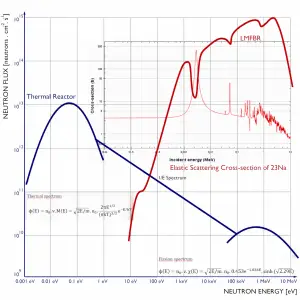
From the physics point of view, the main differences among reactor types arise from differences in their neutron energy spectra. The basic classification of nuclear reactors is based upon the average energy of the neutrons, which cause the bulk of the fissions in the reactor core. From this point of view, nuclear reactors are divided into two categories:
- Thermal Reactors. Almost all of the current reactors built to date use thermal neutrons to sustain the chain reaction. These reactors contain neutron moderator that slows neutrons from fission until their kinetic energy is more or less in thermal equilibrium with the atoms (E < 1 eV) in the system.
- Fast Neutron Reactors. Fast reactors contain no neutron moderator and use less-moderating primary coolants, because they use fast neutrons (E > 1 keV), to cause fission in their fuel.
Types of Nuclear Reactors
The main conventional types of nuclear reactors are:
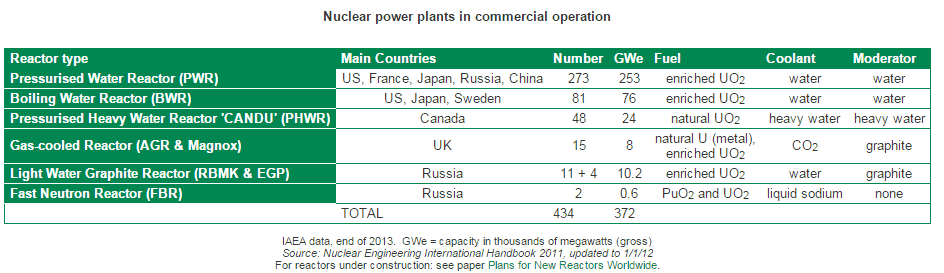
The most common nuclear reactors are light water reactors (LWR), where light water is used as a moderator. LWR’s are divided into two categories:
- Pressurized water reactors (PWR) – are characterized by a high-pressure primary circuit (to keep the water in a liquid state)
- Boiling water reactors (BWR) – are characterized by controlled boiling in the primary circuit
Principle of operation of PWR-type reactor
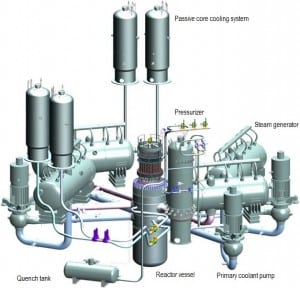
Source: http://www.bellona.ru/
Pressurized water reactors use a reactor pressure vessel (RPV) to contain the nuclear fuel, moderator, control rods, and coolant. They are cooled and moderated by high-pressure liquid water (e.g.,, 16MPa). At this pressure, water boils at approximately 350°C (662°F). The inlet temperature of the water is about 290°C (554°F). The water (coolant) is heated in the reactor core to approximately 325°C (617°F) as the water flows through the core. As it can be seen, the reactor has approximately 25°C subcooled coolant (distance from the saturation).
The hot water that leaves the pressure vessel through the hot leg nozzle is looped through a steam generator, which heats a secondary loop of water to steam that can run turbines and generator. Secondary water in the steam generator boils at a pressure of approximately 6-7 MPa, which equals 260°C (500°F) saturated steam. Typical reactor nominal thermal power is about 3400MW, thus corresponds to the net electric output of 1100MW. Therefore the typical efficiency of the Rankine cycle is about 33%.
See also: Types of Nuclear Reactors
Main Components of Nuclear Reactor
The key components common to most PWR-type nuclear reactors are:
-
The body of the reactor vessel is constructed of high-quality low-alloy carbon steel, and all surfaces that come into contact with reactor coolant are clad with a minimum of about 3 to 10 mm of austenitic stainless steel (e.g.,, 304L) to minimize corrosion. Nuclear reactor core. The reactor core is a bounded region, where a neutron multiplication occurs and where a chain reaction takes place. The reactor core contains the nuclear fuel (fuel assemblies), the moderator, and the control rods.
- Reactor pressure vessel. The reactor pressure vessel is the pressure vessel containing the reactor core and other key reactor internals. It is a cylindrical vessel with a hemispherical bottom head and a flanged and gasketed upper head. The bottom head is welded to the cylindrical shell while the top head is bolted to the cylindrical shell via the flanges. The top head is removable to allow for the refueling of the reactor during planned outages.
- Neutron reflector. The reactor core is surrounded by a neutron reflector or reactor core baffle. The reflector reduces the non-uniformity of the power distribution in the peripheral fuel assemblies, reduces neutron leakage, and reduces a coolant flow bypass of the core.
- Lower support structure.
- Surveillance capsule assembly. The capsules contain reactor vessel steel specimens obtained during vessel fabrication and are withdrawn periodically from the reactor vessel.
- Core support barrel. The core barrel belongs to the lower core support structure because it houses a reactor core.
- Upper guide structure assembly.
Reactor Control
In general, the reactor thermal power and the outlet temperature of the coolant from the reactor core are controlled by manipulating several factors which affect the core’s reactivity. In PWRs, these factors are especially:
- position of control rods,
- the concentration of boric acid in the RCS,
- core inlet temperature.
These three parameters determine the reactivity of the reactor system. A control rod is removed from or inserted into the reactor core to increase or decrease the reactor’s reactivity (increase or decrease the neutron flux).
See also: Reactor Control
Reactor Criticality
The basic classification of states of a reactor is according to the multiplication factor as eigenvalue, which measures the change in the fission neutron population from one neutron generation to the subsequent generation.
-
Reactor criticality. A – a supercritical state; B – a critical state; C – a subcritical state keff < 1. Suppose the multiplication factor for a multiplying system is less than 1.0. In that case, the number of neutrons decreases in time (with the mean generation time), and the chain reaction will never be self-sustaining. This condition is known as the subcritical state.
- keff = 1. If the multiplication factor for a multiplying system is equal to 1.0, then there is no change in neutron population in time, and the chain reaction will be self-sustaining. This condition is known as the critical state.
- keff > 1. If the multiplication factor for a multiplying system is greater than 1.0, then the multiplying system produces more neutrons than are needed to be self-sustaining. The number of neutrons is exponentially increasing in time (with the mean generation time). This condition is known as the supercritical state.
See also: Reactor Criticality
Example – How to Change Power of Nuclear Reactor
During any power increase, the temperature, pressure, or void fraction change, and the core’s reactivity changes accordingly. It is difficult to change any operating parameter and not affect every other property of the core. Since it is difficult to separate all these effects (moderator, fuel, void, etc.), the power coefficient is defined. The power coefficient combines the Doppler, moderator temperature, and void coefficients. It is expressed as a change in reactivity per change in percent power, Δρ/Δ% power. The value of the power coefficient is always negative in core life. Still, it is more negative at the end of the cycle primarily due to the decrease in the moderator temperature coefficient.
Let us assume that the reactor is critical at 75% of rated power and that the plant operator wants to increase power to 100% of rated power. The reactor operator must first bring the reactor supercritical by inserting a positive reactivity (e.g.,, by control rod withdrawal or boron dilution). As the thermal power increases, moderator temperature and fuel temperature increase, causing a negative reactivity effect (from the power coefficient), and the reactor returns to the critical condition. It is necessary to continuously insert the positive reactivity (via control rods or chemical shim) to keep the power still increasing. After each reactivity insertion, the reactor power stabilizes itself proportionately to the reactivity inserted. The total amount of feedback reactivity that must be offset by control rod withdrawal or boron dilution during the power increase (from ~1% – 100%) is known as the power defect.
Let assume:
- the power coefficient: Δρ/Δ% = -20pcm/% of rated power
- differential worth of control rods: Δρ/Δstep = 10pcm/step
- worth of boric acid: -11pcm/ppm
- desired trend of power decrease: 1% per minute
75% → ↑ 20 steps or ↓ 18 ppm of boric acid within 10 minutes → 85% → next ↑ 20 steps or ↓ 18 ppm within 10 minutes → 95% → final ↑ 10 steps or ↓ 9 ppm within 5 minutes → 100%
Fuel Consumption of Nuclear Reactor
Consumption of a 3000MWth (~1000MWe) reactor (12-months fuel cycle)
It is an illustrative example, and the following data do not correspond to any reactor design.
- A typical reactor may contain about 165 tonnes of fuel (including structural material). A typical reactor may contain about 100 tonnes of enriched uranium (i.e., about 113 tonnes of uranium dioxide).
- This fuel is loaded within, for example, 157 fuel assemblies composed of over 45,000 fuel rods.
- A common fuel assembly contains energy for approximately 4 years of operation at full power.
- Therefore about one-quarter of the core is yearly removed to the spent fuel pool (i.e., about 40 fuel assemblies). At the same time, the remainder is rearranged to a location in the core better suited to its remaining level of enrichment (see Power Distribution).
- The removed fuel (spent nuclear fuel) still contains about 96% of reusable material (it must be removed due to decreasing kinf of an assembly).The annual
- l natural uranium consumption is about 250 tonnes of natural uranium (to produce about 25 tonnes of enriched uranium).
- The annual enriched uranium consumption of this reactor is about 25 tonnes of enriched uranium.
- The annual fissile material consumption of this reactor is about 1 005 kg.
- The annual matter consumption of this reactor is about 1.051 kg.
- But it corresponds to about 3 200 000 tons of coal burned in coal-fired power plants per year.
See also: Fuel Consumption
Material Problems and Challenges of Nuclear Reactors
The main problems or rather challenges, that must be taken into account when designing reactors are:
- Pressure and temperature stress with associated limits
- Pressure and Temperature (P/T) Limits
- Heat up and cooldown rates
- Low-temperature Overpressure Protection Limits
- Pressurized Thermal Shock
- Radiation Damage to Reactor Materials
- Corrosion
See also: Material Problems of Nuclear Reactors
Special Reference: Reactor Pressure Vessel Status Report, U.S. NRC. NUREG-1511. Office of Nuclear Reactor Regulation U.S. Nuclear Regulatory Commission, Washington, 1994.
Manufacturing of the Reactor Vessel – Youtube
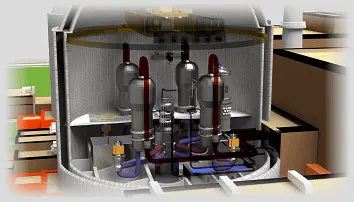
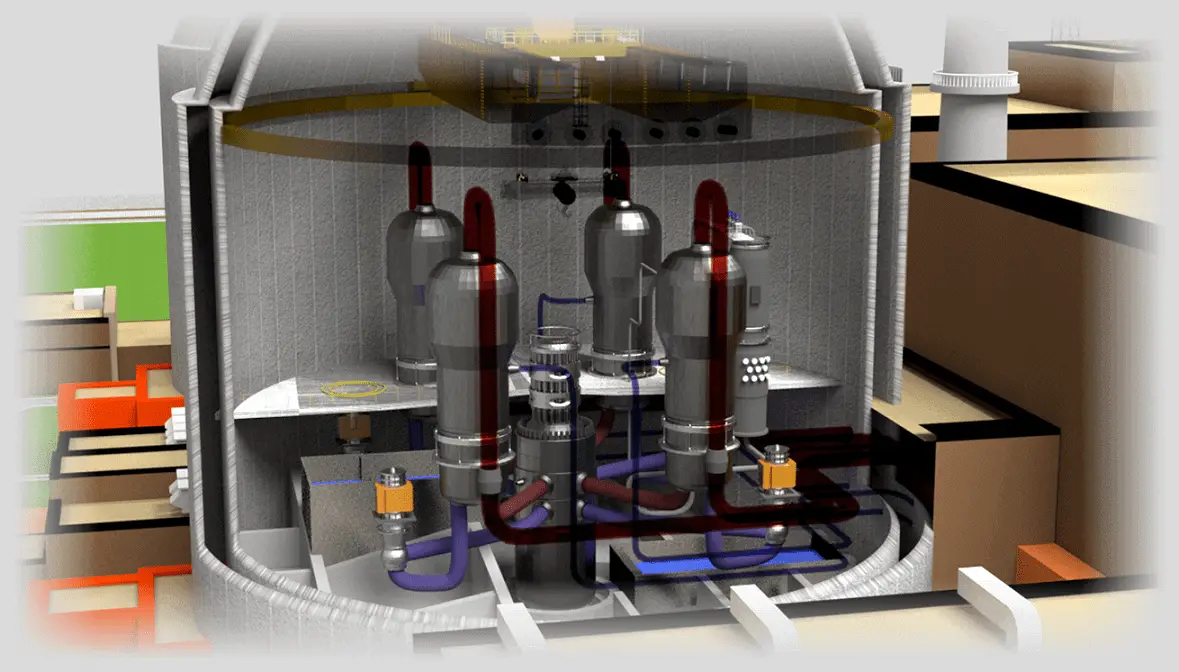
Did you know?
The world’s first nuclear reactor operated about two billion years ago. The natural nuclear reactor formed at Oklo in Gabon, Africa, when a uranium-rich mineral deposit became flooded with groundwater that acted as a neutron moderator, and a nuclear chain reaction started. These fission reactions were sustained for hundreds of thousands of years until a chain reaction could no longer be supported. This was confirmed by the existence of isotopes of the fission-product gas xenon and by different ratios of U235/U238 (enrichment of natural uranium).
The existence of this phenomenon was discovered in 1972 at Oklo in Gabon, Africa.